New drugs for flu, bees read electrical fields, why moles are sensitive to seismic vibrations and how good is a sharks sense of smell...?
In this episode
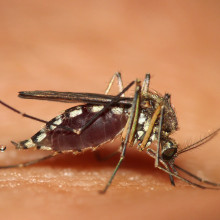
01:08 - Mosquitoes become desensitised to DEET
Mosquitoes become desensitised to DEET
Mosquitoes become less sensitive to the common repellent, DEET (N,N-Diethyl-meta-toluamide), after continued exposure to the chemical.
DEET is a widely used mosquito repellent. and has been shown in various studies to repel up to 100% of mosquitoes. But a new study, published this week in PLoS ONE, suggests that repeated exposure to the chemical can temporarily reduce the insects' aversion to it, potentially leaving a person vulnerable to bites and mosquito-borne diseases, like malaria.
Nina M. Stanczyk and her colleagues at Nottingham University exposed female mosquitoes to a human arm that had been treated with DEET. Any that approached on the first trial were removed.
The remaining mosquitoes were then presented with the DEET covered arm again. This time, around 50% of them were no longer repelled by the chemical.
The same was found when a heat source was used in place of the arm, and even when the first exposure was to DEET with no arm or heat source present.
This study is interesting, as the mosquitoes can't just have learned to overcome the DEET because it was associated with a reward. They weren't given a blood meal in any of the conditions, and the effect occurred even when the first exposure was to DEET alone, with no attractive stimulus along-side. This means it is most likely to be due to processes called "sensory adaptation", or a related phenomenon called habituation.
This occurs in humans too. If you walk into a room where there is a powerful smell it can seem overpowering at first; but, as time passes, you notice it progressively less as your nose "gets used to it". This is because of sensory adaptation. Habituation is similar, but is down to attention. Rather than specific sensory neurons becoming adapted, it relates to the fact that if we sense something over and over we become bored of it, and stop paying so much attention to it. Either of these mechanisms could be responsible for the reduction in the mosquitoes' sensitivity to DEET.
To find out what was causing them to become less sensitive to the DEET, the researchers made recordings of electrical signals from the insects' antennae. The mosquitoes' that had become less sensitive to DEET at the second exposure also showed a reduced signal in response to the chemical.
This study is important when it comes to testing new repellents, as if they are tested repeatedly on the same insects, their effectiveness may decline. It is also extremely important to find out if this is going to cause problems in tropical countries, where repellents are used to prevent the transmission of diseases like malaria.

05:09 - In Utero MRI
In Utero MRI
The brain is a hugely complex organ made from expansive networks of cells, all connecting and communicating with one another, quickly and effectively. This vast array of connections begin to form in utero, when the brain is rapidly growing and developing, and when the foetus is extremely sensitive to any kind of disturbance. In a paper published yesterday in the journal Science Translational Medicine, a team of scientists and doctors from the Wayne State University in Detroit outlined their ground-breaking work, developing a way to monitor the development of connections in the brain in the growing foetus.
The team used a variation of the non-invasive MRI brain scanning technique to assess connectivity in the brains of 25 foetuses. The technique, called resting-state functional magnetic resonance imaging, measures the magnetic differences between oxygenated and deoxygenated blood flowing through the brain. Active parts of the brain require more oxygen in the form of oxygenated haemoglobin in the blood, compared to inactive brain regions. The difference in oxygenated and deoxygenated blood can be detected by the MRI machine and can give us crucial information about where functional connections in the brain are being made, both at rest and during a variety of tasks.
The foetal scans demonstrate that brain connections are made during the second and third trimesters, between 24 and 38 weeks into pregnancy. Not only were the group able to successfully scan the moving foetus (not a small feat!) they were also able to show that connectivity gradually increases as the foetus grows. Scans at this stage of development have previously only been possible in premature babies, under situations where the brain may have been altered as a result of preterm birth. Of particular importance was the finding that many connections are made between the two sides of the brain, the cerebral hemispheres. Although the use of the technology in this way is in its infancy(!) the potential for effective and safe foetal monitoring is clearly a big step forward.
The value of these findings is emphasised by supporting evidence from other labs that show a variety of neuropsychiatric conditions, such as ADHD, schizophrenia and post-traumatic stress disorder, may be caused by alterations to the connectivity of the brain. As these connections appear to be developing in the foetal stages of life it may become possible, using this technique, to identify and correct the abnormal connections being made in the brains of patients suffering from conditions such as these.
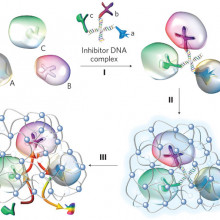
09:17 - Synthetic enzyme organelles
Synthetic enzyme organelles
Scientists in the US and China have invented a way to encapsulate teams of enzymes in a thin polymer shell. This enables the enzymes to carry out a series of sequential reactions within an enclosed space, as happens in nature.
The first step is to identify inhibitors - molecules that will specifically attach to a given enzyme. A number of different inhibitors - each specific to its own enzyme - are then linked by a string of DNA. In the presence of the different enzymes, each inhibitor hooks its own enzyme, trapping them around the DNA scaffold and creating a complex.
Each enzyme complex is then wrapped within a thin layer of a polymeric network using an in-situ polymerisation technique. Gentle heating removes the DNA scaffold and inhibitors, leaving the functioning enzymes encased within the polymer and creating, in effect, a synthetic organelle.
The researchers illustrated the utility of their system by sobering up drunken mice with a packaged enzyme complex that metabolises alcohol. Inebriated mice treated with the complex sobered up more quickly than untreated mice.
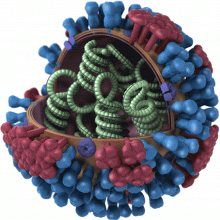
14:54 - Flu-fighters: new influenza agent found
Flu-fighters: new influenza agent found
A potent new flu drug has been discovered by scientists in Canada. It works by deactivating an enzyme called neuraminidase. This is present on the surfaces of flu virus particles where it has the role of breaking down a sticky substance on cells called sialic acid, which would otherwise prevent the virus from spreading to surrounding uninfected cells.
Drugs called neuraminidase inhibitors, famously Tamiflu (oseltamivir), already exist to target this system.
They work by binding tightly to the same part of the enzyme that recognises and cuts sialic acid, preventing the enzyme from working.
But their use is limited because resistant strains of flu appear quite quickly when these agents are used, since the virus can alter the shape of its neuraminidase enzyme to prevent the drug from binding.
Now University of British Columbia scientist Stephen Withers has taken an alternative approach. He reasoned that if a drug is almost identical in shape to the molecule the enzyme is supposed to work on, then it is much more difficult for resistance to develop because a change in shape to avoid binding the drug would also prevent the normal molecule binding too.
So Withers and his team have come up with a family of molecules based on a chemical called difluorosialic acid (DFSA).
This closely resembles the normal sialic acid recognised by neuraminidase, but when the 'flu enzyme acts on it, the resulting chemical change produces a molecule that binds and blocks the enzyme, deactivating it for up to 100 hours.
In tests on different strains of flu, the new agents repeatedly outperformed Tamiflu and its competitor Ralenza (zanamivir), including against strains of the virus that were already resistant.
The new compounds also blocked the growth of flu in cells infected in the dish, critically without producing any signs of toxicity in the cells themselves, and tests on flu-infected mice protected all of the animals from what was otherwise a lethal dose.
As the scientists say in their paper in Science, the similarity of these new drug molecules to the molecules that are already naturally present in the body makes them "attractive antiviral candidates"...
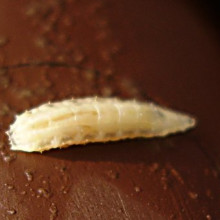
18:03 - Fruit flies defend eggs using alcohol
Fruit flies defend eggs using alcohol
Fruit flies protect their eggs by laying them in alcohol-laced fruit, but only when there are parasitic wasps around, new research has revealed.
So-called endoparasitic wasps lay their eggs in the larvae of other insects. After they hatch, the wasp larvae then live off the fly larvae, devouring them from the inside out.
Infected flies combat the threat by switching to a diet of fruits that are naturally much richer in alcohol, which has the effect of killing off the wasp developing inside them. But now scientists have shown that they also sidestep these parasites in another way.
Emory University researcher Balint Kacsoh and his colleagues have shown that, when there are parasitic wasps around, adult fruit flies prefer to lay their eggs in alcohol-rich food sources, achieving significantly better survival rates as a result.
It seems that flies have evolved to recognise when their offspring will need to self-medicate with alcohol, and so lay their eggs in a food source that leaves them in the best position to do so.
But when there are no wasps around, the flies choose lower-alcohol locations for their eggs and achieve even higher survival rates.
The flies identify the wasps by sight, the team found. Flies genetically engineered to be blind did not switch to the alcohol-laden fruit when the wasps were around.
The memory of spotting a wasp also remains with the flies for some time, and they continue to prefer alcohol-rich sites for the eggs for around 4 days after each wasp encounter. This suggests they form long term memories and use these to determine laying choices.
In further tests, flies engineered to lack a protein needed for long term memory formation reacted normally when exposed to wasps, but didn't retain the memory like normal flies did - they switched back to laying in the non-alcoholic food as soon as the wasps were no longer around.
Interestingly, the flies could also tell the gender of the wasps, and only switched to the alcoholic laying dish when there were female wasps in the area, as only female wasps can lay eggs, and therefore harm the fly larvae.
There are some kinds of wasp that lay eggs on fly larvae, and others that only infect the fly once it has started to pupate. There is no way for the adult fly to protect her offspring from the wasps that infect the pupae because the larvae are likely to have moved from their original food source by the time they pupate. Cleverly, the flies were able to tell the difference between these two kinds of parasitic wasp, and only lay eggs in alcohol when the larvae-infecting wasps are around.
So if you are a fruit fly larvae at least, a little booze can definitely be a good thing!
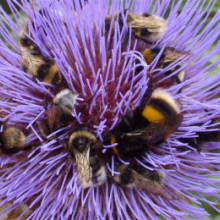
22:00 - Sparks fly between bees and flowers
Sparks fly between bees and flowers
with Professor Daniel Robert, University of Bristol
We've long known that many animal such as hammerhead sharks and platypus can read the electric field of their prey. But new research out this week suggests that bees might also be able to sense electric fields around flowers. We spoke to Professor Daniel Robert from Bristol University to find out more.
Chris - So first of all, what did you do this for? Why were you going looking at bees and electric fields?
Daniel - The idea was to first look at adhesion in pollen grains because we were interested in the travel of a pollen grain from the flower to a bee and the fact that it has to stick to a bee. And then when the bee visits another flower, the pollen grain has to jump from that bee to the next flower for pollination to take place. So, we started with that and soon realised that the electricity or electric fields, electrostatics was actually very important in the life of that pollen, in the transport of that pollen. They soon became apparent that this electricity was not just because the pollen had a charge on it, but that was also because the bee, as it approaches a flower carries a charge.
Chris - So then you say, right, the bees got a charge. So, the flower also has a charge. So, is the bee going to be sensitive to that?
Daniel - So, as a sensory biologist, at first, my question was immediately, can the bee pick that up? Does the bee know that there is a charge difference or a potential difference between herself and the flower? o, it was known from a literature that bees carry a positive charge as they move across the air.
Chris - Is that just because the bees are rubbing against their molecules as they fly?
Daniel - That's correct, because the air is ionised very often, except for when there's a lot of water, that cancels out the ionisation. When the air is sufficiently dry, airplanes or helicopters also have the same effect, they charge up as they move through the air.
Chris - Do bees get an electric shock when they land?
Daniel - No, they don't because they accumulate that much. They can accumulate a charge equivalent to 100 or 200 volts or so, not thousands of volts as airplanes or even ourselves can accumulate when we walk on static carpets for instance.
Chris - So, what happens when this bee with its net positive charge approaches a flower then?
Daniel - Well, the important bit in that is that the bee is in the air, so it's not earthed to anything, so it carries a charge by itself. So, it's kind of a little bundle of positive charges and it approaches the flower which is itself then linked to the ground. It's earthed. And because flowers are mostly made of water and water is a conductor, flowers will tend to be at a potential or the type of level of electricity if you want, that is on the Earth. So, as the bee approaches with a positive charge, the flower is relatively negative to it. So there wouldn't be an effect where plus and minus will generate an electric field in between these two objects. So, as the bee approaches even without touching the flower, there is a force being developed there.
Chris - Does it matter whether it's a flower or other bits of the plant because after all, petals are just modified leaves, aren't they?
Daniel - That's correct. We haven't looked at petals necessarily so far, but your question is right. There is no reason to think that petals might not be of the same effect.
Chris - So, what effect do you think this has on the bee?
Daniel - So, what we could show is that bees can learn the presence or the absence of these charge difference in the flower. So, we designed a learning experiment for bees in the lab for bumble bees in which we presented artificial flowers which where all of the same colour and all had little pads underneath, electric pads in order to test whether the bee could tell or whether there was a charge or not. And what we did, we repeated experiments that people did before in studying, learning about bees is that we produce some flowers that have sugar and some flowers that have quinine. So, bees like sugar and they don't like quinine. So, on the flowers that had sugar, we put 30 volts of voltage bias which is very reasonable. We find that in nature and the others were at 0 volts, and we soon discovered that after 40 or 50 visits, individual visits by individual bees, that we know, we marked. Bees will tend to choose the flowers with the sugar with 80% accuracy.
Chris - By presumably recognising that there's that field, so they must be sensitive to it. How do you think they're using that in nature, if at all?
Daniel - Well, when we remove that field after the learning experiment, we took the same bees that were then educated to find the sugar associated with the electrical charge. When we removed that, bees were unable to find the flowers with sugar. So, they have used that field to orient themselves.
Chris - How far away from a flower are they sensitive to, do you think? Do you think this is actually a useful thing for them in nature to orientate themselves, locate food sources, and maybe also avoid visiting flowers more often than they should before the nectar is replenished?
Daniel - That's right. We think that the bees can detect that at 4 or 5 cm or so from say, a petunia flower and the functionality of that, as you suggest, is about the nectar and the pollen. What flowers want to do is advertise themselves as much as they can to bees. They have nice scents, they have nice colour, they have a texture, they have asymmetry. They look beautiful to us, but they look fantastic to bees of course. Now, what we show is that when a bee visits a flower and we could measure that with electrodes that we implanted into flowers, the potential of the flower changes. And when a bee visits that potential changes and when the second bee comes along, and happens to land for instance, that potential changes even more. So, it would be in the interest of a bee to understand that perhaps that flower has been visited and is depleted from nectar. Therefore, not incurring the risk of predation by spiders, but not wasting time in foraging about.
Chris - Calling at a shop that's already been visited.
Daniel - That's right.
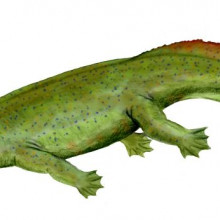
29:16 - Redesigning tetrapods - Planet Earth
Redesigning tetrapods - Planet Earth
with Jenny Clack, University of Cambridge, Stephanie Pierce, Royal Veterinary College
360 million years ago, the first four legged creatures started moving from the water onto land. But what did our (very) distant ancestors look like?
Early research suggested they were something like a salamander. But new studies of the fossils, carried out by Jenny Clack from the University of Cambridge and Stephanie Pierce from the UK's Royal Veterinary College, has found that these 'tetrapods' were nothing like the pictures in textbooks.
Planet Earth Podcast presenter, Richard Hollingham, went to see one of the fossils and talk to Jenny and Stephanie at the Zoological Museum in Cambridge. He started by asking Jenny about the assumptions they were challenging...
Jennifer Clack: The first creatures to emerge from the water and start to evolve legs with fingers and toes, people assumed that these would have had legs with weightbearing limbs and that they would have walked in the same way that modern four limbed creatures walk, like a lizard perhaps or a salamander, and they envisaged these early tetrapods, as we call them, as giant salamander-like creatures and that's how they were first reconstructed.
Richard Hollingham: So, Stephanie, what did you do? What did you do to these fossils to find out what they were really like?
Stephanie Pierce: Well over the last three or four years what we've been doing is we've been looking at the fossils that are still embedded inside the rock, so the fossils that we can't see on the outside and to do this we've been using modern technologies, we've been using micro CT scanners and when we looked at the vertebral column the bones of the vertebrae that were still trapped inside the rock we weren't able to identify all the different parts that were supposed to be there based on what had been described in the literature and in text books over about the last 100 years and so this got us really, really puzzled. What was actually going on in the backbone of this animal? And what we decided to do was take it to the European Synchrotron Radiation Facility and create UB high resolution images using synchrotron x-rays and what we found was something very, very interesting.
Richard Hollingham: So you've got a representation of this; what you saw inside this fossil on the computer screen in front of us and it's a three dimensional representation and this wasn't what you expected.
Stephanie Pierce: It's not quite what we were expecting. When you talk about modern tetrapod backbone their vertebrae are composed of individual units that are all interconnected and those units are composed of only one bone. An early tetrapod vertebral column is actually slightly different. It's actually composed of many different bones and in particular each unit is supposed to be composed of three separate bones, it is supposed to be composed of one bone in the front, which is called the intra centrum and that's the green bone in the image here. One bone on the top which is called the neural spine and that's the pink bone in the image and then a pair of little bones at the back called the pleura centra which are the yellow bones in this image. But that sort of concept of what an early tetrapod vertebrae looked like isn't actually what we found when we did the synchrotron scanning of this fossil.
Richard Hollingham: So what did you find? What was different about it?
Stephanie Pierce: Well, some of the bones ended up being fused together and in particular the bone at the front, the intra centrum, was actually fused onto the back of the pleura centra and this was very unusual because that indicated to us that the first bone in the series, the intra centrum, was actually in fact the last bone in the series and this meant that all the literature in the text books previously actually had the backbone back to front in these animals.
Richard Hollingham: What does this actually tell you about the animal - what it looked like and maybe how it moved?
Jennifer Clack: One of the things that we found you can see represented by a series of blue elements and the nearest equivalent we can find in modern animals is the sternum, the breastbone, and that corresponds to the idea that these creatures were not walking with alternating footprints as you would expect but actually were using a kind of crutching motion. So using their front legs to, kind of, hop forwards and then pushing or stabilising with the back legs because the back legs were paddle shaped, they weren't walking legs, they didn't have proper knees or ankles, they were stiff and probably were just sitting there in the mud and used for swimming in the water but as stabilisers and anchors on land.
Richard Hollingham: So based on this study what do you now know that they look like?
Jennifer Clack: It's shoulders were relatively enormous and it had this big rib cage which then faded out into a rather weak bit of backbone just before where the hind legs fit in, and the hind legs were paddles set sort of vertically against the body and a little short stubby tail, a big head, big shoulders and rather diminutive hind quarters.
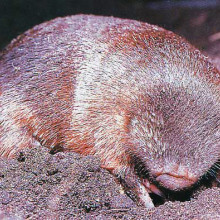
34:51 - Subterranean hearing
Subterranean hearing
with Dr Matthew Mason, University of Cambridge
Animals from poor visual enviroments can evolve incredibly good hearing. For example, the nocturnal Tawny Owl can pinpoint the direction in which a mouse is scurrying within 0.01 seconds. But what about those animals who live underground? Ginny Smith was joined by Dr. Matthew Mason from Cambridge University whose work looks at the middle ear bones of subterranean mammals.
Ginny - So, can you tell me how the middle ear bones work in humans.
Matthew - So there's three parts to your ear. The outer ear is the bit that you can see, you've got the earflap and the ear canal, and if you were to go down your ear canal, at the bottom of it, you'd find your eardrum which is a very thin membrane. And the idea is that when sound comes in down your ear, that membrane will vibrate. Now, on the other side of the membrane is the middle ear cavity and that's an air filled space, one in each of your ears in the middle of your head. And sound has somehow got to cross that air filled cavity from the eardrum into the last part of the ear which we call the inner ear, and within the inner ear, we've got the hair cells and these are specialised cells with little hair-like processes. When the fluid inside the inner ear vibrates then the hair cells will turn that into electrical signals that the brain can understand. So, the bones that I look at are bridging the gap, if you like, between the eardrum and the fluid filled inner ear - three little bones which have to conduct the vibrations.
Ginny - And why do we need them?
Matthew - Well, the problem is that your inner ear is filled with fluid and this evolved in vertebrates a long time ago perhaps in aquatic vertebrates. And if you're aquatic, there's no real problem because sound is traveling in water. It can go straight into the fluid filled inner ear, it can vibrate the hair cells, and you can hear it. But when tetrapods moved onto land then suddenly, sound was reaching them through the air, and the problem there is, that if sound comes in through the air and it hit fluid, just like the fluid in your inner ear, most of the energy, 99 % or so will be reflected straight back off. And you can get an idea of that effect if you're in the bath, if your head is underwater, someone tries to talk to you, it's very difficult to hear what they're saying because most of their energy has simply bounced back. So, the middle ear device that we have, the eardrum and the three little bones, the purpose is to help to translate more of that energy from the air into the inner ear so we can actually hear it. And it's what physicists call impedance matching. But it means that much more of the energy actually gets through. It gives us a better chance to hear sound that's coming to us through the air.
Ginny - Okay, so that's how it works in humans. What's different for animals that live underground?
Matthew - Well, the problem if you live in a tunnel underground is what kind of sounds you're going to be receiving and people have done studies where they've played sounds in tunnels underground and it tends to be low frequencies that travel best. But even low frequencies don't travel very far. They only travel a few meters before the energy tends to be absorbed by the tunnel walls. So sound really doesn't propagate very well and then to make matters worse, many subterranean mammals are solitary. They live on their own, in their own tunnel system. The next neighbour is going to be in a completely separate system and then how would you communicate between yourself and your neighbour? So, two problems - sound doesn't travel very far and the tunnel systems may not be connected. So hearing perhaps is not terribly useful to you, but ground vibrations might be a better thing to detect because if you can produce vibrations in the soil or if something is producing vibrations in the soil, they can travel between the burrow networks and they seem to travel better. They can travel further.
Ginny - So, how have their ears changed in order to pick up these vibrations?
Matthew - Well, it depends on the group, but one of the groups that I'm interested in is called the golden moles and these live in Africa. These little guys are interested in vibrations that might be made by prey species for example. Now, what they've done with their ears is in essence, they've turned them into little seismometers. So, the middle ear bones in us, in humans and in mammals in general are usually very, very tiny and they need to be tiny in order to transmit sound vibrations effectively. But in the golden moles, these bones have become very, very large in some of the species. And so, we've got this disproportionately massive ear bones. Now, let's put this in context. The Namib Desert golden mole that I look at is about the size of a mouse, but its ear bone is twice the size of a humans. Now, that perhaps doesn't sound too impressive, but if you imagine the mole blown up to the size of us, it would be like us, having a solid lump of bone, the size of your fist in each of your ears.
Ginny - Wow! That doesn't sound very comfortable.
Matthew - It isn't but it might be useful for detecting vibrations. So, the idea is that if you had happened to be touching the ground the ground was vibrating, then your whole head would tend to move, but these great lumps of bone will tend to stay in one place. And that would give you some relative movement and then that could go to the hair cells, the hair cells could pick up those vibrations and suddenly, you've got an ear that can detect ground vibrations. You've got a seismometer.
Ginny - So, does that mean that these moles have to literally bury their heads in the sand in order to detect vibrations?
Matthew - They absolutely do, yes. So, the Namib Desert moles have two ways of getting around. They can burrow through sand, but the problem with sand is you can't actually make a burrow system because it just collapses behind you. So, if you're burrowing underground, you've always got your head in contact with the sand. And in fact, they use their head for burrowing anyway so it's always in contact. But sometimes these little guys like to run around on the surface which is perhaps less energetically costly, they can go further, it's easier to do it. But if they run around on the surface, they've got this problem that they're not touching the ground. But what they do every couple of feet is they stop, they actually push their heads into the sand and listen.
Ginny - And how far can they hear?
Matthew - Well, we don't know that, but we do know that they seem to be able to locate grassy tussocks where their prey lives, at distances of up to about 20 meters. At least they seem to know where the tussock is and they head towards it.
Ginny - Brilliant! Well, that sounds fascinating, but I can't imagine walking around with two fist-sized lumps of bone in my head. I think I'll stick to our version of hearing.
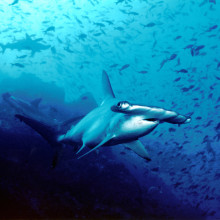
41:37 - How well can sharks smell?
How well can sharks smell?
with Dr Jonathan Cox, University of Bath
Sharks sudden appearance at shipwrecks has historically credited them with a sniffy supersense. We wanted to find out whether new research into exactly how smell reaches a sharks nostrils confirmed the legend so Chris Smith spoke to Dr Jonathan Cox from the University of Bath to find out more.
Chris - So, tell us about your work then. You actually started with a shark in a tank, but it wasn't a live one?
Jonathan - That's right. It was a plastic shark or rather a shark's head that we'd made a model of as a result of getting a real shark's head from the Natural History Museum into a CT scanner and getting the scanned data and turning into a plastic model which we then stuck into a tank in an engineering department.
Chris - So, you take the real shark, scan it to get very high resolution, ultrastructure or what makes up that head, all of the passages. So, you can see the structure and then you make a model so that you're going to do experiments on the model?
Jonathan - That's right, yeah and we made, as I said, a plastic model, but most of it was opaque and a little section of it which comprise the nasal region was transparent so we could see into it.
Chris - And this means that then you can begin to ask questions very consistently like, how does water flow around this when it's moving along, how does water flow along the nasal passages and all that kind of thing I presume?
Jonathan - That's right, yeah. So, in order to do that, we used tiny filaments of red dye and fired them at the transparent nasal region, and looked to see what happened.
Chris - So, you didn't make the head move. You made the water move because obviously, it's the same thing. The water is going to flow the same way whether the head is moving or the water is moving, and then you're tracing where those filaments of dye go.
Jonathan - That's right. It's much easier to make the water move. Getting a head to move is pretty difficult. Although it was a hammerhead shark and the head of a hammerhead shark wobbles from side to side, so hammerhead sweeps its head from side to side. So, it would be nice actually to mimic that motion and that's a sort of a future experiment.
Chris - So, hammerheads have their eyes on the ends of the hammers. Do they also have their nostrils there then too?
Jonathan - Yeah, they're right on that front edge, sort of in the face of the action as it were. So, the eyes are on the sides and the nasal region is right on the front edge of the head.
Chris - So, what happens then when in your case, the dye, but say this were a shark out in the open ocean and there was some chemical in the water that it was sniffing down, a blood of a prey for example. What's actually the process?
Jonathan - Several things happen. First of all, water enters nasal passages and gets distributed over the large sensory surface area that's got packed into its nasal chambers. And as a result of getting past over the sensory surface get sensed. But also weirdly, quite a lot of the water or flow actually passes over the head and we think that this actually helps paradoxically flow to go through the nasal chamber, so it helps the flow through the nasal chamber.
Chris - What about the fact that those nostrils are so far apart? How does the shark exploit that? I mean, it must have evolved that way for a very good reason and this presumably does give it an enormous advantage of sniffing down concentration differences.
Jonathan - Yes. Well, it's still that the further apart your two noses are or two nostrils are, then the easier it is to locate the source of a scent. So yeah, that's what it seems to be, but it has not been proven.
Chris - So, going back to where we started which was that people say, sharks have this extraordinary ability to track down prey over enormous distances. Do your experiments bear that out?
Jonathan - That's a physiological experiment which we were not able to do, working with a plastic model, but what we were able to do is to show how the flow - in other words, the water carrying a scent is distributed over the sensory surface area within the nose.
Chris - So, once it actually goes up the nose, what happens then because there is some quite interesting studies done on humans showing that we close off one nostril at one time and have a relatively open nostril, and this changes the rate at which the fluid air in our case (it will be water in the shark obviously) flows over the surface that detects things because some things are more quickly detected by the respiratory - the ciliated surface that does the detecting. So, the more water or the more fluid that can go up there and the more odorant, the more you're going to smell while other chemicals take longer to dock on to the things that smell them. So, slowing down the flow a bit is beneficial. Do you see the same thing in these sharks?
Jonathan - Possibly with a hammerhead because it sweeps its head from side to side. One swivel of the head, it's going to encounter odiferous water. In other words, that's got the odorant molecules in and the other side, the other nose as it were is going to be kind of blocked to use your terminology because it's not going to be in the scent, but then it all comes back again. And also, by shaking the head from side to side, it's possible that there's a little bit of agitation of the water inside the nasal cavities and a kind of asymmetric mixing of that water. None of that is proven, but that's something to be looked at.
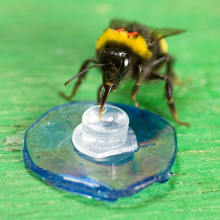
47:40 - How do bees see flower iridescence?
How do bees see flower iridescence?
with Alison Reed, University of Cambridge
Recent studies have shown that bees can recognise iridescence. This is the rainbow pattern that you see on the shiny side of a CD and some plants use iridescence to give them their colour too. Kate Lamble headed over to Cambridge University's Bee Laboratory to speak to PhD student, Alison Reed about her research.
Alison - I'm particularly interested in looking at flower colour and the texture of a flower surface to see how bees and insect pollinate and interact with that surface.
Kate - Why do you think your research is important? How can it help us understand the natural world?
Alison - Well, because pollination and insect behaviour is so important and it's all around us, and it affects a lot of the things that we value including food production. That just a general understanding of how this process works is really valuable and it might feed on to other projects which might be able to benefit crop production and food production in the future.
Kate - So, how do bees see the world? Is it particularly different from what we see as humans?
Alison - Well, the colour spectrum that they see is very different to us. So, they can see UV wavelengths which we can't see and the colours that they see are quite different to what we see. And so, we need to look at things from the bee's point of view and do experiments to see if they can see colours that we can see basically. So, we're looking at iridescence in flowers because even though we can see it, bees may not be able to see it, so that's why we need to test it.
Kate - When I think of iridescence, I think of really shiny things like beetles or butterflies, but I don't necessarily think of flowers. Can you tell us a little bit more about how it works in plants?
Alison - It's very similar. A lot of the structures that animals use are also used by plants, but it wasn't really studied until very recently and that's why our lab is really interested in it. For example, we're mainly looking at flowers which have diffraction grating, so they have grooves, they're all folding at the cuticle on their petals, and that produces a diffraction grating which makes iridescence. I mean, that does happen in animals, but mostly, animals use multi-layers, but petals predominantly use diffraction gratings. It's fairly unique to flowers and we haven't found any flowers which have multi-layers on their petals.
Kate - If you want to test whether a bee can see these tiny folds on a flower, how do you go about testing that?
Alison - Well, the folds on a flower, very similar to the folds that you find on a CD. So, when you rotate a CD, you'll see the different colours and the iridescence, and that's exactly the same structure that we see on a flower. So sometimes to begin with, we wanted to see if they could see that CD iridescence because it's a perfecting grating. So, it produces a perfect iridescence. So, we first would test to see if they could see that and they could. And so then we followed that up by doing some experiments with flowers which had similar structures but not quite so perfect and they could also see those diffraction gratings in that iridescent effect.
Kate - Are there any particular flowers that you use that are particularly iridescent?
Alison - One of the first ones that we discovered was tulip flowers which have quite a good iridescence obviously and you could see that's why, one of the reasons why they're so shiny when you look at the surface of a tulip. And we've used those for most of our experiments because that's what's been used in the past, but over time, we're gradually finding more and more flowers that have got even better diffraction gratings and even better iridescence, so hopefully, we'll test them with those in the future.
Kate - If you're testing to see if a bee can see that iridescent effect, how do you physically go about it? Do you just show a bee your flower and see what happens?
Alison - Well, we can't really use real flowers because there are so many other things to consider like scent, so we make artificial flower in the lab out of plastic, and we do a differential conditioning experiment where you have two different flowers - one is iridescent and one isn't. Then with the non-iridescent flower, you provide a punishment which is a bitter quinine solution and with the iridescent one, you provide a reward which is a sugary sucrose solution. But if they can tell the difference between the two, they should learn not to go to the quinine and the horrible tasting flowers, but to go to the iridescent ones.
Kate - Could you show me a couple of those artificial flowers that you've got?
Alison - Yeah, of course. This is one made from a CD, so you have an impression of the CD and dental wax which makes a mould basically, a really fine structure mould and then you can make a plastic replica of the surface using resin.
Kate - So, it sort of looks like a small plastic coin with a hole in the middle.
Alison - It's basically like a miniature CD with a pigment in the plastic resin.
Kate - And why is there a hole in the middle? What's that used for?
Alison - That's used for putting a sucrose reward so the bees can feed from the middle, so it's basically replicating the structure of a flower.
Kate - It doesn't look particularly like a flower. I mean, if it was me, I'd make them to look like pretty petals.
Alison - The most important thing for us is that they're all the same so that they're comparable. I guess we could make them look like petals, but these bees have never seen flowers before so it wouldn't make much difference, I don't think.
Kate - If you've made that one from a CD, how would you go and make one from a flower?
Alison - It's very similar. You have to make sure that the petal that you're casting is very flat and you do the same thing basically. So, you go into the gardens and you find a flower that you're interested in testing and you make up the wax and you press the petal into the wax, and it dries within 10 minutes and then you've got your cast, your mould for making your discs.
Kate - So, what have you found out? Is there a difference between the CD ones that you've been using and the tulips?
Alison - It's very similar for CDs and for flowers, but bees move faster between the iridescent disks than they do between the non-iridescent ones. So, when you're using flower casts made from flowers with flat cells, that produces a very different result to flowers that have this diffraction grating on their surface.
Kate - So, do you think that flowers have evolved these tiny structures in order to attract insects like bees?
Alison - Sort of co-evolved I would've thought, insect vision and flower colours sort of co-evolved. I mean, it's important for the flower that it's unique in its environment so that it gets pollinated because obviously, it wants pollen from its own species to be transferred to another flower of the same species. So, it's really important that these flowers are unique and unusual. And that's one of the reasons probably why this is so helpful for flowers because it makes them unique.
Kate - Has there anything come up that you haven't expected?
Alison - How temperamental the bees are I think. You don't realise how much they're affected by the weather and by the conditions that you keep them in, and just what they feel like on a day to day basis I guess. So, sometimes you'll get bees that come and start an experiment, and then they'll go back to the colony and they won't appear for another few days and your experiment has been ruined. So, you have to start from the beginning again, but I guess that's all part of working with animals.
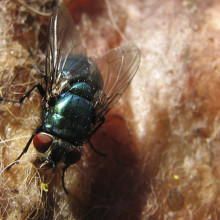
54:15 - From how far can flies smell food?
From how far can flies smell food?
Marcus - Hi. I'm Marcus Stensmyr from the Max Planck Institute for Chemical Ecology in Germany.
Yes, it's true. A fly could smell meat over that distance, although only under very, very favourable conditions.
"Mark-release-recapture" experiments performed with house flies caught at a poultry farm showed that flies could find their way back to the farm from a distance of up to 7 km.
To get this data, the scientists had to tag and release 160,000 flies, of which only 0.05% were recaptured.
So, flies can do it, but far from all will manage this feat.
Seven kilometres is not only a considerable distance to smell something, for a tiny fly, simply traveling 7 kilometres is a major effort, taking several days.
Even so, flies are able to detect a smell of rotting meat over long distances. The exact distance depends on many factors such wind conditions, size of the meat and landscape features.
For flies to smell something 7 kilometres away, it nevertheless has to be a substantial piece of meat like a herd of dead elephants rotting away in the Sun, or an entire poultry farm.
How do flies manage this? Well, it involves no magic - simply, a very good sense of smell. Flies like all other insects detect odours with their antennae which are densely colour in hair-like structures containing olfactory sensory nerve cells. These nerve cells are extremely sensitive to volatile chemicals, odours that is. And in the case of flies that feed on the dead and decaying flesh, so-called carrion flies, their olfactory cells are also optimised towards detecting cadaver odours...
- Previous How do pills know where pain is?
- Next Addicted to Love?
Comments
Add a comment