We explore the basis of brain diseases on this week's Naked Scientists.& We find out what happens to the brain in Huntington's disease, discover the genes behind Alzheimers and a potential treatment for autoimmune diseases like Multiple Sclerosis or MS.& Also, the nerve cells in the ear that make loud sounds painful, the extraordinary eyes of the Mantis Shrimp and the world's largest web spinning spider.& Plus, how spiders make glue from silk and snot, and in Kitchen Science, we show you a way to fool your brain into making your body do something unexpected.
In this episode
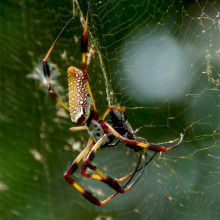
World’s biggest web-spinning spider
Halloween is approaching, and what better story to get us in the spooky mood that the discovery of the world's largest web-spinning spider.
The record breaker, named Nephila komaci, is a type of golden orb weaver spider from Africa and Madagascar. The female have bodies up to around 4cm long (1.5 inches) and their legs reach to 12cm: so she would stretch out across the palm of your hand. The males are much tinier, about 5 times smaller than the giant females.
Tarantulas still hold the title of king of the spiders. The Goliath bird eating tarantula from South America has a leg span of 30cm and inch-long fangs! But they don't make webs.
This giant web-spinner is the discovery of Matjaz Kuntner from the Slovenian Academy of Sciences and Arts and Jonathan Coddington from the Smithsonian National Museum of Natural History in Washington DC, publishing in the online journal PLoS One.
And for a long while they thought this spider that was first found in 1978 must be extinct, or perhaps a hybrid of two other species, because only that single specimen was known. Together these two researchers went on expeditions to South Africa but they failed to find a living specimen of this giant spider. And even searching through 2500 more specimens from 37 museums, still another one didn't show up.
Then eventually, another specimen turned up from Madagascar, and three more were found in South Africa, confirming that this is indeed a new species.
That adds another name to the list of around 41,000 spider species that are currently known to science (every year another 400 or 500 are added).
While obviously being an important discovery for spider biodiversity, these spiders also shed light on how and why some female animals evolve to be much bigger than their male partners. In their paper, the spider sleuths build a family tree of the known species of Nephila spiders, showing that there is a branch of African spiders in which the females evolved to be bigger and bigger over time.
The discoverers of this fantastic new species are urging others to go and try and find more of these spiders, because they are obviously extremely rare and they want to know more about them. The only place they are definitely known to live is in the sand forests of Tembe Elephant Park in KwaZulu-Natal in South Africa.
A rare wonder indeed and a treat for Halloween...
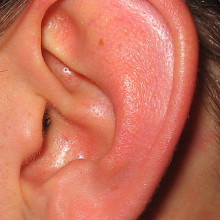
Painful to listen to
We've probably all had cause to use the phrase "painful to listen to", but now scientists in the US have discovered why. Writing in Nature, Johns Hopkins researcher Paul Fuchs and his colleagues show that a small group of nerve cells in the ear respond to sounds that would best be described as "traumatic". The ear converts sound waves into brain waves using a specialised structure called the cochlea. Here, vibrations of the eardrum are transferred to a group of cells called hair cells. The information from these hair cells is then transmitted to the brain by a family of nerve cells. 95% of these nerves are known as type I fibres and respond very strongly to even the quietest sounds. But the other 5%, which are known as type II neurones, have remained something of an enigma, because, in previous attempts to study them, they didn't seem to respond to sounds at all.
Now, the Johns Hopkins team have shed some light on the mystery by managing to record, in young rats, the electrical activity from inside some of these unusual nerve cells. They also labelled some of the cells with a dye so that they could study their structure and how they were wired up to the cochlea. What this has revealed is that the cells are activated by an excitatory nerve transmitter chemical, called glutamate, which is released from the sound-sensing hair cells. But the recordings suggest that the sounds have to be very loud indeed to trigger any response, meaning that the cells might be there to help to process and discriminate different types of very loud sound. The team also found that the nerve fibres respond to another nerve chemical called ATP, which is often associated with tissue damage, so they could also be providing the brain with a way to monitor the health and function of the of auditory system. This means that they might help us to better understand and even treat hearing problems like tinnitus, which causes sufferers to experience distracting high-frequency buzzing noises.
According to Fuchs, "no one thought recording them was even possible," he said. "We knew the type II neurons were there are now at lest we know something about what they do and how they do it."
Why Mantis shrimp eyes work better than DVDs
The most sophisticated eyes in the animal kingdom belong to mantis shrimps a group of extraordinary species that live on coral reefs around the world. In a brand new paper just out, a team of scientists examine just what lies behind these complex eyes and they've uncovered some tricks of nature that could find applications in the cutting edge of modern technology, perhaps spawning a new generation of DVDs and CDs.
Last year, a paper in the journal Current Biology announced the discovery that mantis shrimp can see both linear polarized and circular polarized light. Now a team led by Nicholas Roberts from the University of Bristol have discovered that mantis shrimp eyes do this using special light-sensitive cells that act as a device known as a quarter-wave plate. Essentially these plates convert circular polarized light into linear polarised light. Manmade quarter wave plates are vital components of DVD players and some camera filters, but they don't work nearly as well as the ones that have evolved in the eyes of mantis shrimps.
Normal light behaves like a wave that can vibrate in any direction. In contrast, linear polarized light only vibrates in only one plane. We usually don't see polarized light, although we are aware of it, for example the light that reflects off glass or the surface of a pool of water (putting on polarizing sunglasses cuts out those polarising rays and lets you see through the water).
Circular polarized light behaves like a spiral or a helix. The remarkable mantis shrimps can even tell the difference between circular polarized light spins to the left or to the right.
Not only that, but unlike manmade quarter wave plates that only work well in one colour, mantis shrimp eyes work almost perfectly across the whole visual spectrum from near ultra violet to infra-red.
Leading on from their earlier work, the research team discovered that the receptors that detect circular polarized light are located in a central band across the mantis shrimp eye - their eyes are divided into 3 distinct regions, giving them trinocular vision in each eye and are packed with light-detecting units called omatidia, similar to other invertebrates including insects. They did this essentially by shining polarized light of different types through thin sections of parts of the eye and measuring how well those cells absorbed the light.
Part of the reason why these biological structures work so much better than manmade structures is because they are so tiny - on the nano scale - with components that are smaller than the wavelength of even ultra violet light (10-400nm). That is very challenging to create in manmade structures.
The big question is why on earth do mantis shrimps need such incredibly complex eyes?
There is lots of polarized light bouncing around the clear shallow waters of coral reefs where most mantis shrimps live. Their favourite prey includes small slivery fish whose scales polarize light.
Scientists also think that mantis shrimps might use circular polarized light as a way of secretly communicating with each other. Parts of their bodies reflect circular polarized light, and we so far don't know that any other animal can detect this type of light. So perhaps they send out signals - flashing mating colours maybe - without any fear of being detected by anyone else except another mantis shrimp.
So when it comes to solving technical problems like how to see well in a bright watery world, natural selection has come up with an elegant and simple solution. And perhaps the mantis shrimp will teach us a thing or two about how to build the optical devices of the future.
12:01 - Engineering Spider Glue
Engineering Spider Glue
with Dr Randy Lewis, University of Wyoming
Scientists have identified what it is that can make spider webs so sticky, and the genes that spiders use to actually make them. Knowing this could bring us a step closer - maybe even a spider step - to making our own spider-based glues. Joining Chris Smith to explain how is Randy Lewis from the University of Wyoming...
Randy - Hello.
Chris - Welcome to The Naked Scientists. Tell us. So how are you, first of all, identifying these genes that spiders use to make their webs so sticky?
Randy - Well we took webs, about 100 of them actually, and washed the glue off of those webs; then we separated the proteins that make up that glue, and, using some chemical tricks, we were able to get some evidence of the proteins that were in there. And then we did a mass spectrometry study of all the peptides to find those and then used that information to go back to the spider itself and identify which were the genes that were involved in making these spider silk proteins.
Chris - I see. So because we know the genetic code, and we know what the protein sequence is that's in the spider stickiness that we've washed off the web, we can work out what gene sequences probably went into making those proteins? So if we then go back to the spider spinneret I guess - the structure that makes a silk - and ask, "can we find any genes like what the sequence would be in there?" then you got a chance of finding them?
Randy - Right. And especially in this case, because all the glue comes from a specialised gland. So you can actually just go directly to that gland and not worry about other kinds of genes because the predominant genes that are being made or being used in that gland are going to be for the spider silk glue.
Chris - And, presumably, the glue isn't just one particular protein. It must be a cocktail?
Randy - In this case, we believe it's two proteins actually and one of them looks more like a silk protein, the regular silk protein. The other one actually looks like what we call a mucin protein which makes up slime and snot. So, our combination is, is that it's really a silk and snot protein and the two of them together provide both strength and stickiness.
Chris - I think spiders will probably be mortified if they realize that you were calling their web stickiness as snotty. But is it possible to do what the spider does in its backend, in a test tube? In other words, can you borrow from biology? Can you copy this effectively?
Randy - We're in the process of defining whether we can do that or not, but we believe we can because the proteins are actually very simple and we need to find a system that can reproduce that. And we believe that if we can get those genes into some insect cells that grow in culture, that those cells should be able to produce the proteins with the sticky parts on them. The key here is, is that one of the proteins in particular has a whole lot of sugars put on it and you need to be able to have those sugars, we're fairly sure, in order to be able to get the stickiness. So we believe that using something like insect cells to start with, we can reproduce what the spider has and then actually test the material and see how well it performs.
Chris - And that's presumably because insect cells, evolutionary speaking are much closer to a spider than say, one of our cells would be. And so therefore, they're likely to have the right chemistry going on in the cell to add those sticky sugar molecules.
Randy - Right. And also, it turns out that insect cells are fairly easy to work with. So, we think that in inserting the genetic code from the spiders, also probably will fit better with the insect cells.
Chris - And if you are successful in making this happen, what will you be able to do with this glue?
Randy - Well, right now, we're not exactly sure, but we think that there certainly are possibilities for some biomedical applications, for closing on sutures, things like that, other places you might be able to use glue. We're also hopeful, and that remains to be seen, that you can use it in something like Epoxy and that is, put the two components separately won't be - won't give you that the real stickiness and that when we put the two together, you'll have a glue, and that's also very useful in a number of applications. And so, you can basically put it together and then have it be sticky and separately, they're just fine.
Chris - And lastly Randy, I understand that you are currently heading across Canada to rescue some goats. What's that all about?
Randy - Basically, a company we worked with, NECSI Biotechnologies, developed some transgenic goats that make the spider silk proteins in the milk and the company has - for all intent and purposes, gone under. And so, we're right now about 20 miles from the farm and this afternoon, we're going to go and prepare the goats. We'll pick them up tomorrow morning and bring them back to Wyoming, so we can preserve the genetics of those goats that have been made, because the company can't afford to keep them anymore. And we're going to move them down to Wyoming and keep the genes going and actually, use the milk to produce protein now or we can really get serious about looking at various kinds of products from it.
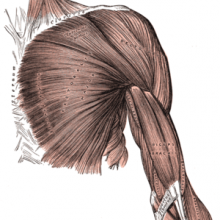
18:01 - Kitchen Science - Rising Arms
Kitchen Science - Rising Arms
with Ben Valsler & Meera Senthilingam
Ben - I'm sorry to say that this week, Dave Ansell is actually in bed with a flu, leaving me to handle Kitchen Science all on my own. But luckily, I'll be joined later on by Meera Senthilingam who will help me demonstrate an old experiment that I've known about since I was very little where you can fool your brain into making your body do something very strange. It's a really simple one to try out at home, so do please give it a go. All you need is a doorway and your arms. So, find the doorway, stand in the middle of the doorway, facing in to the room and drop your arms down by your side. Then press the backs of your hand doorframe and push and push and push and push. You need to push for about a minute. It'll start to hurt, it'll feel very uncomfortable, but you need to keep going. And after a minute, all you need to do is step forward and relax your arms.
....
Ben - Welcome back to Kitchen Science. Today, I'm having to cope without Dave but I do have a very willing victim. Sorry, volunteer, Meera Senthilingam. Hello, Meera.
Meera - Hello.
Ben - Now, you've seen this experiment done before, haven't you?
Meera - Yeah, but a while ago.
Ben - So then you might have an idea to what's happening, but hopefully will still be a bit of a surprise. So if you could come with me over to this doorway then we can get you set up.
Meera - Okay.
Ben - So, you need to stand in the doorway, facing into the room. Now this door is actually quite narrow which is kind of perfect for us because you don't want to have to lift your arms too much. If your door is a lot wider then it might work better to get somebody to put their foot behind the door and just make the right size space between the door frame and the door itself. But here, there's maybe 6 inches maybe either side of your hand, so that's pretty much perfect. You feel comfortable standing in the doorway?
Meera - Yeah. As comfortable as you can be in a doorway.
Ben - And now the next thing to do is put your arms down by your sides and then just and place the backs of your hands against the doorway. So they're level with your hips and pushing out on the door.
Meera - Okay.
Ben - Now, here's the tricky bit. What I need you to do now is to push outwards on the door frame, as hard as you're comfortable with and you're going to have to do this for about a minute. So, when you're ready, start pushing.
Meera - Okay.
Ben - Well I can see from your face that you are genuinely putting some effort in here. Now the thing with this is initially doesn't feel too bad. You're just pushing out against the doorway and nothing really feels like its happening. But then after, probably about this much time, it actually starts to get quite uncomfortable. But don't stop. Do keep pushing. How are you feeling?
Meera - Uncomfortable, but I'm trying. I'm trying. I'm concentrating.
Ben - Well we still have to go for a little bit longer yet so I may as well distract you by explaining what's going on in your brain. Now your brain sends messages to different groups of muscles, telling some to relax and some to contract in order to create the movement that you need. Right now, certain muscle groups will be contracting as hard as they can to try and push out against the door. But obviously, they're not doing very well unless you have a very flimsy house. In which case, you may have broken your own doorway. How are you feeling now?
Meera - It's quite painful actually and is it okay that my arms are actually shaking a little bit?
Ben - The shaking I think is just evidence that you're really putting in the effort to it. I think you've probably had long enough now. So what I need you to do, when I say go, I need you to step forward and just relax your arms completely. So, are you ready?
Meera - Okay.
Ben - Go.
[Meera's arms mysteriously rise up from her sides - without her trying to do so]
Meera - My arms! Okay. That was quite cool.
Ben - And you do look a bit like you're sleep walking now as your arms appear to be stuck out in front of you. But how does it feel?
Meera - It feels completely normal. Like it doesn't feel that my arms have raised at all. It just feels that they're just dangling and I can actually just happen to see them in front of my face.
Ben - And normally, if were to hold your arms out in front of you, it would actually take some effort. But does this position feel like you're totally relaxed and this is where your arms are supposed to be?
Meera - Yeah. Basically, as if I was in a swimming pool or something and my hands were just sitting on top of the water. That's what it feels like.
Ben - Well, what's actually happening to your arms has, until very recently, been a bit of a mystery. Back in the 1920s, people knew about this experiment but they assumed that it was all to do with the spinal cord. Because there are nerve clusters that control the length of muscle and so they assume that the spine was involved. But now, in a very new paper that came out in September in the journal Brain Research, Amy Parkinson and her colleagues have actually done this very experiment inside a brain scanner to have a look at what's going on. Now they compared scans of people making this movement on purpose, to people doing it exactly how we've just done, pressing against something for a while and then this involuntary movement happening afterwards.
If people had been right in the '20s and it was a spinal cord only issue, then you'd see very little brain activity or certainly nothing significant compared to normal. But what they found was significant brain activity in the areas that were concerned with making and planning movements. And they found this activity just before the movement happened. So this wasn't a response to the fact that your arm was moving of its own accord. This really was the effect that made it happen. They also did find some activity in the brain that happened after the movement and this suggested that that was a response to the fact that your arm was involuntarily moving.
One of the bits they did find quite a lot of activity in is called the cerebellum. Now this is what's responsible for motor learning and error corrections. So it must have learned while you were pushing out. That your arm was apparently supposed to be shorter than it really is and that's why the muscles contracted and your arms rose up at their own accord.
This is very similar to an experiment that we did a little while ago on The Naked Scientists where we put special prismatic glasses on. These shift your vision 10 degrees to one side and then when you try and throw and catch a ball, initially, you're totally useless until your cerebellum kicks in and corrects for the fact that when you thought you'd thrown it in one direction, your eyes tell you you're throwing it in a different direction. After a little while, you can throw and catch because the cerebellum has done its job.
Meera - I actually remember having a go on that around the office and it did adapt really quickly. I was really surprised.
Ben - It's strange and frustrating when you take the glasses off again and you find that you can't throw and catch for a little while.
Meera - Yeah, but I'm not amazing at that any way, so it's alright.
Ben - Hopefully, Dave will be back in action for another Kitchen Science next week.
We'd like to say huge thank you to Dr. Ellie Dommet at the Open University and Professor Patrick Haggard at UCL for helping out with this week's experiment.
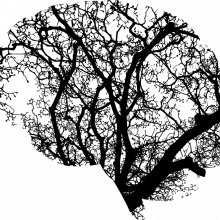
Why do neurons not regenerate?
Well, the answer is that the brain's neurones have an architecture that's what's called post-mitotic: there are only a few restricted areas in the brain and central nervous system where there are new nerve cells being born in an adult brain.
This means that, for the most part, you must rely on the complement of nerve cells that you are born with - and which continue to divide for a very short window after you were born - meaning that what you're born with is what you have to make last a lifetime.
There's a reason for this, because if brain cells were dividing all over the place - and remember that brain cells have long connections that they make from one cell to the other, and those connections are crucial to you being able to do the right thing, say the right thing, have memories and for your brain to be able to work properly - if those cells were dividing all over the place and making aberrant connections, then it will be very, very difficult to preserve that architecture. So there's kind of method in the madness.
The problem is that, as that is a fixed structure, it's very hard to repair it by getting the cells to re-divide because basically, if you have an injury that's bad enough to destroy a part of your brain or your nervous system, evolutionarily speaking the chances are you'd be dead anyway. So, we haven't really evolved the ability to repair the brain and spinal cord.
In some animals though, that can happen and things like gold fish, lampreys, and also even salamanders can restore whole limbs, and bits of their nervous systems. If you take the eye out of a frog, turn it around and put it back in again, it will rewire itself back into the brain, only, because the eyes now are upside down, the animals see upside down and it does the wrong thing. If you hold a fly in front of it, instead of jumping forward at the fly, it jumps backwards and takes a bite out of the deck.
That won a Nobel Prize for Roger Sperry a few years ago and proves that some animals can regenerate their nervous system, but certainly, not us unfortunately.
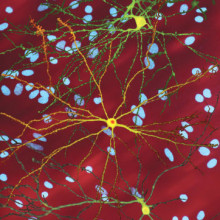
21:17 - Huntington's and Immunity
Huntington's and Immunity
with Dr Ed Wild, University College London
One of the most important neurological diseases is one called Huntington's disease. It affects about 8 in every 100,000 people here in the UK. It's a genetic disorder and people tend to get the disease symptoms by the time they're aged about 40 or 50. It affects a person's ability to move and also impairs cognition and behavioural processes, especially in the later stages of the illness. Research is on-going currently to try work out why Huntington's disease occurs, why people get the symptoms they do and whether we can reverse, or at least arrest the process. One of the scientists studying Huntington's disease is Ed Wild; he's based at University College London's Institute of Neurology. He's interested in how the disease affects the functioning of the immune system, and what role that might play in the disease. He spoke to Chris Smith about this work, beginning with the genetics of Huntington's disease...
Ed - We're quite lucky in Huntington's disease research because the genetics is quite well worked out and we've known what the gene is that causes Huntington's disease since 1993, after a massive international research collaboration to look for it. And that in many ways, puts us at a bit of an advantage over other brain disease like Alzheimer's which we'll be hearing about later on, motor neuron disease, Parkinson's disease where there are genes that are known to influence the disease. But in Huntington's disease, we know exactly what gene causes it. It has two names. One is the Huntington gene and the other is IT15 which stood for Interesting Transcript-15 which is perhaps the genetic understatement of the '90s. And this is a gene that encodes for a protein called Huntingtin. So the gene is the recipe for this Huntingtin protein. We've known about this now for 16 years. We know a lot of things that a Huntingtin protein does but it's still a very mysterious protein. It's a very big protein and it's one that's very difficult to work with.
Chris - Do we understand at all about why it is that it causes the very discreet symptoms it does? I mentioned some of them. People with Huntington's first of all tend to notice that their movements go a bit array and then they start to get other symptoms as well and this takes up to 40 years before it manifests itself. So why is that and why are there these very discreet changes to people's movements and behaviour?
Ed - Well, what we do know about HD is that the bit of the brain that's affected earliest, at least as far as we can tell, looking at it under microscopes or with brain scans, is the basal ganglia. And those are the sort of deep grey matter structures down in the brain that have very important stop-go and coordinating functions for movements. And so, one of the characteristic features of Huntington's is a phenomenon called Chorea which is from the Greek word for dancing. That's where we get the word choreography from. And that's because patients with HD almost invariably get these unusual dancing like movements, involuntary movements of the arms and legs, and face. On top of that though, they get problems with voluntary movements. They lose their voluntary movements, a bit like what you see in Parkinson's disease.
Chris - Is this because they're actually physically losing brain cells? And if so, what's going on in the cells that are dying? Why are they dying?
Ed - Well, that's the million dollar question for HD families. We know lots of things are going on, but at the moment, there's no clear consensus on what the most important function of the Huntington protein is, that makes the cells die. What we're certain about though is that the cells are unhappy or dysfunctional for many years before they die. And that's good news because it means if we can reverse that dysfunction, we could potentially prevent patients who we know have the mutation from going on to develop the disease. But exactly, you know, what the processes are that are going on in the cells, we know that there are lots of them. But, exactly what the balance of problems is, is unclear at the moment.
Chris - Interestingly, not all brain cells seem to be vulnerable to the same extent though, do they? So do we know why some cells seem to perish and others are less affected?
Ed - No. that's a really important question and as I say, we know that the cells of the basal ganglia, the striatum are selectively involved early on. What's weird though is that those aren't necessarily the cells where, under the microscope, you see the most accumulation of the abnormal Huntingtin protein that's seen throughout the brain, but the striatum doesn't seem to display a lot of that. And there are various theories as to why this might be. Probably, the most popular theory is that those cells receive a lot of inputs from other areas of brain. So those are incredibly busy cells, very metabolically active cells and they're connected to a lot of other cells, and it may well be that there's a phenomenon called excitotoxicity going on where the cells get too many inputs. And that tips them over into the balance of not being able to cope because they were unhappy already because of having the abnormal protein floating around. But there's another theory which is that - and this goes to the heart of the mutation that causes HD - It's unlike other mutations where it's a single spelling mistake. You know, changing one DNA letter to another. This is a genetic mutation in which one word, one three-letter word, CAG is repeated again, and again, and again, too many times, and that causes the protein to take on an abnormal shape. And the striatum does seem to contain cells with even more abnormal repeats than the rest of the brain and that may be one reason why it has this selective vulnerability early on in the disease.
Chris - And looking outside the brain, you're interested in the immune system because of course, this gene isn't just turned on the brain. It's turned on in other cells in the body too. So how does it affect immune function?
Ed - Well, that's right. The gene and the protein have been found to be expressed basically everywhere that they've been looked for. And what we did was to look in the blood of HD patients. We're looking for biomarkers, things that can be used to measure from the outside, what's happening to a patient's brain on the inside. And we need, basically, accessible tissues. You can't go diving into someone's brain so we look in blood to see if we can find changes due to the gene. And what we found quite surprisingly, we weren't really looking for it, but what we stumbled on almost was a signature of immune activation that the cells, that the blood of HD patients contains a signature of cytokine proteins that suggests that the patient is in a sort of chronic inflammatory state. The immune system is overactive and we did a bit of detective work to try and figure out what the relationship was between the gene that causes the disease and the cytokine production. And we think that we've identified that it's actually the white blood cells which are expressing the gene and that in some way makes them over active. They become hyperactive and we've detected that in the blood. Meanwhile, our collaborators in Washington and the US have been looking at expression of these cytokine proteins in brain and found that they're over-expressed in HD brain as well. So there seems to be a sort of commonality between the brain and the blood there.
Chris - And just to finish Ed, does that mean then potentially that some of the pathology could be because the immune system is attacking cells and making the situation worse or it could be triggering the cells to become diseased or is this just literally a red herring? The immune system has these useful predictive markers that tell you what the state of the brain is, but they're not in themselves bound up with what's going on with the brain.
Ed - I think at the very least, it suggests that these might be useful as markers, but I think - to be honest, I think there is more to it than that because a number of people have looked at trying to adjust the immune system in HD in the brain. And have produced some very promising results showing that if you can damp down certain pathways involving the microglial cells, the immune cells of the brain, you can produce quite a dramatic survival effect on HD mice. So it seems that the microglial, immune cells of the brain are acting as policemen which are having a useful effect early on in the disease but later on as sort of becoming a rather unruly bunch of riot policemen, hitting innocent civilians in the face and doing more harm than good.
30:07 - Damping Down Multiple Sclerosis
Damping Down Multiple Sclerosis
with Professor David Wraith, Bristol University
Helen - Multiple sclerosis or MS and it's a condition in which nerves in the brain undergo a process known as demyelination. This is where a layer that surrounds nerve fibres called the myelin sheath which protects nerves and helps them to transmit information becomes damaged. And this in turn can lead to all sorts of symptoms that include impaired movement, vision, sensation, and cognition. And the cause of the damage to the myelin is immune system. For some reason, it fails to tell friend from foe and begins to attack the body's own brain tissue. This is known as autoimmunity. But it's been known for 100 years that we can desensitize the immune system by presenting it with tiny doses of the thing that it's overreacting to. And doctors use this trick today to treat patients with allergies to things like peanuts. More recently, researchers at Bristol University have found that it can be used to damp down MS and also reduce some of the damage that it does. Ben Valsler went to meet Professor David Wraith to find out more.
David - What we've realised is that by designing fragments of the target for attack in the autoimmune disease, we can focus our attention on a subset of immune cells called the T cells. And as such, you can avoid the risks of anaphylaxis or autoimmune disease exacerbation by focusing in on the parts of the antigen that the T cells recognize. And we have shown that administration of these peptide fragments can lead to desensitization by the allergic and autoimmune state in experimental models that we'd run in the laboratory. And we recently expanded this or extended this into clinical trials in man.
Ben - Usually, with a vaccine against the pathogen, something like the flu, you would administer a dead version of the virus and that would present the surface proteins to your immune system and that means you're immune system can be primed to attack these surface proteins when it recognizes them. So you've been able to isolate the active part of the proteins that only the T cells react to. And this means that you don't get the overblown immune reaction that could lead to anaphylaxis.
David - Actually, it'sis more subtle than that because the other thing we've learned is that the immune system is actually a fine balance between what we call effector cells that are designed to battle against the pathogen. But also, the sort of military policemen who regulate those soldiers that are out there, battling against the pathogens. And what the military policemen are there to do is really to sort of dampen down and control the immune system. I mean, when I was talking about this to a BBC reporter some years ago, it was around the time of the first Gulf War and he said to me, "You know, autoimmunity sounds to me just like friendly fire. It's where the immune system is designed to fight off enemies, but occasionally, things go wrong and it starts attacking its own folks, right?" And in a way, that's true. But what we've now learned since then, is that there are other mechanisms designed to dampen down those, sort of, pathogen clearing T cells. And if you look in parasitic infections, the cells we are designing our vaccines, are peptides, to induce are in fact present there and are preventing the immune system over-attacking the response to the parasite. And this is why one of the mechanisms that has allowed parasites to evolve, to live along with man.
Ben - So whereabout are you in the trial so far?
David - We just conducted a small phase one strike two clinical trial and the regulatory authorities asked us to conduct this in patients suffering from quite severe MS already. So one wasn't expecting any dramatic change in the condition with these individuals. Really, when you do a safety trial, you're primarily trying to prove that the approach you are taking and the treatment is safe and well-tolerated. And indeed, this was the case. There was one individual in the trial who was suffering from severe loss of visual acuity and almost was clinically blind in one eye. And through the course of the treatment, her vision was clearly recovered and this is very, very encouraging. Obviously, one has to do much more extensive trials to prove that this is a more general phenomenon. And of course, knowing what we know about multiple sclerosis, I think everybody would accept that this is just the type disease why you need to start treating as early as possible. So our next trial will be a much more extensive trial in patients with an earlier stage of disease.
Ben - Increasingly, with medicine, we're looking at treatments for an individual, knowing that everybody is genetically unique. Genes we know code for proteins and it's parts of proteins that you're hoping to encourage the immune system with. So, could it possibly be that everybody will respond differently and this may be an enormous task of finding exactly which bits of which genes code for which bits for which proteins in order to successfully make a vaccine?
David - Well, I think that's one of the points about autoimmune diseases that we really do understand. There's a genetic contribution to these diseases. But actually, of that, the major genetic contribution or predisposition to disease comes from a set of genes called the human HLA genes or the histocompatibility complex genes. And these are the genes that code for proteins that are the receptors for the fragments that we're talking about. So in fact, whereas something around 25% of the population express a particular HLA molecule that we're targeting, around 70% of MS patients carry that receptor. So indeed, our vaccine, if you wish to call it that, would be expected to be effective in about 70% of the MS sufferers in the UK at least. But in fact, there is a good deal of cross-binding or cross-reactivity between the particular HLA molecules that we are targeting. So one way to expect it to be effective in over 90% of people.
37:01 - The Genetics of Alzheimers
The Genetics of Alzheimers
with Prof Julie Williams, Cardiff University
Helen - Now, Alzheimer's disease is the most common form of dementia, mostly in the elderly, and a great deal of research is underway to understand, treat, and try and prevent Alzheimer's. But there are currently no effective treatments for it. Now Professor Julie Williams and her team at Cardiff University recently discovered a pair of genes that seem to be link to Alzheimer's. And she joins us now. Hello, Julie.
Julie - Hello, Helen.
Helen - Thanks for coming along on the show. First of all, just what causes Alzheimer's?
Julie - Well, Alzheimer's is caused by genetic and non-genetic factors. We know that there are three genes already that contribute to and cause rare forms of Alzheimer's disease and one gene that increases risk of developing the common form of Alzheimer's disease. And that's the position we were at before we publish our research.
Helen - And do we know what's going on inside the brain when people have Alzheimer's? What's the problem there?
Julie - Well, we know there are certain markers of the disease. So, you see in the brains of Alzheimer's cases, plaques that are made up of what we call beta-amyloid mainly and these occur outside the dying brain cells. Within these brain cells that are dying, you see tangles made up of microtubules. So those are the two markers there. But what exactly is going on is still a basic mystery for us.
Helen - So we have these plaques of beta-amyloid and that's a type of protein, isn't it?
Julie - Yes.
Helen - Yes and do we have any idea now, how these genes now might be involved in those markers, those plaques that we're finding in the brains of people with Alzheimer's?
Julie - Okay. The rare forms, the forms that are contributed to by single gene defects, these occur in genes known as APP and the pre-sinilins, these we know affect the amount of beta-amyloid that occurs in the brain. But what we don't know is, is that the same process for those with the more common form of Alzheimer's disease? And our results will actually tend to support a different, slightly different, process involved in common Alzheimer's disease compared to the rare forms that we've known about for some years.
Helen - So what do we think is going on in that common form of Alzheimer's?
Julie - Okay. What our research has shown is that we identified two new genes that increased risk of developing common Alzheimer's disease. The genes are clusterin and PICALM. And when we put our data are studied together with a French study. We now have definitely three new genes and an additional fourth new gene. And this is opening up new ideas about what actually causes the more common form of Alzheimer's disease.
Helen - And how did you find that association? Did you go out and look at people who got Alzheimer's and look at the genes they have and compar that amongst the population and discover these, sort of, unique genes that might lead to the condition?
Julie - That was exactly right. But it was quite a large experiment and we looked at the genes of 4,000 individuals with Alzheimer's disease and compared over half a million individual variations in each of those individuals, and compared those to 8,000 individuals without Alzheimer's disease. So this is the biggest study of its type, currently published.
Helen - And do we have any idea what these genes normally do and what might be going wrong in the people who got Alzheimer's?
Julie - Okay, some of these genes are involved in a theme that's developing in this program of inflammation which surprised us to some extent. So, the compliment receptor gene, clusterin, is involved in protecting the brain through the inflammatory response, the classical compliment system. And what this is telling us is that inflammation is a primary event in the disease production. We had known for a number of years that you see markers of inflammation within the brains of Alzheimer's individuals. We thought this was secondary to the amyloid plaques for example. But this appears now to be a primary element in disease production. The other element is cholesterol. We see a lot more genes involved in cholesterol and sterol, and we really don't what they are doing but we do know that they are playing a crucial role in disease development.
Which Arm is stronger?
The reason for this is that the nervous system is wired up, so that you balance out movements. so that if a muscle gets made longer than it thinks it should be, you have an organ called a spindle, which is inside the muscle, which signals length of the muscle and if that gets stretched it feeds back on to the mototr nerve supplying the muscle and increases the firing of the motor nerve-making the muscle get a bit shorter. So if you have 2 antagonistic muscles, one hand and the other hand, pulling against the other then one's going to win a bit and that's going to make the muscle on the other arm get a bit stonger then that will pull back and that one will win a bit and make the muscle on that side pull harder and so on. And it will just do a tug-of-war so you won't go anywhere because the brain is set up so you're finger's in the middle.
Does Stress affect memory?
We posed this question to Julie Williams from the University of Cardiff...Julie - Alright. I think it probably is a general problem. Learning, you probably need to have well activated nerve cells to create the synapses to learn new information. So I think this probably could be affected by stress. So I would say it's a general problem. Chris - Because one other thing that people have realized is that the hormones that make our stress cortisol, there are receptors for those chemicals in the brain, and they do seem to cause damage if they're present chronically to those parts of the brain, particularly the hippocampus which is concerned with making memories. So maybe that's part of the manifestation, Julie. Julie - We also know that people who have had major depression throughout their lifetime or bipolar disorder tend to have a highly - a slightly higher risk of developing Alzheimer's disease at later life. This could be due to the medication they take or it could be due to the actual process of depression. So there are some areas of support for that.
Can stem cells treat brain diseases?
We posed this question to Ed Wild from University college London...Well it's a big question and the answer is that there's a lot of promise in stem cells, but we're probably several years away from being able to see the benefits of the research that's going on. You mentioned at the beginning of the program that brain cells, once they're dead, they're gone and they can't be replaced from within the brain because brain cells don't divide. And the hope of stem cell research in neurodegenerative diseases is that you can take these stem cells which are capable still of dividing and becoming any kind of cell they like, put them into the brain and they'll then re-grow, and replace the cells that have died. But as you also mentioned earlier on, the brain is a phenomenally complex thing and performing its functions normally, depends not just on the cells being there but on the connections, the billions and billions of connections that there are between the brain cells. And even if you could get the brain cells, the stem cells to differentiate into neurons that behave completely normally, you'd probably never be able to get them to make all the right connections. So there's certainly be a lot of work, training the stem cells to make the right connections and behave the same way as the cells around them.
Is the blood-brain barrier real?
Absolutely. People talk about this blood-brain barrier. This notional structure which in some way keeps the brain isolated, cocooned inside you biochemically and physically-away from what's happenign in you blood stream and it;s absolutely true. The history of the blood brain barrier goes back a hundred and something years to a guy called Paul Ehrlich, who was a German scientist, he was interested in dyes initially. He used to inject dyes inot animals and then see which bits of the body got stained. andhe was intrigued to see that when he put dyes into the blood stream, much of the time the dye did not get into the brain. And so he realised there must be some kind of barrier separating what goes round in the blood stream from the delicate issue inside the brain. We not understand more about what this blood-brain barrier is. It's a bit contrived, what what's going on is that you basically have special junctions between ceels that line the surfaces of the brain, that separate the brain tissue from blood vessels and these cells make very tight junctions, that's what they're called, and this effecttively means that there's a barrier which is the membranes of those cells separating what's in the blood stream from what's in the blood tissue. And what this means is that certain substances can move very easily into the brain, especially if they're substances that can dissolve well in fat, because of course the membranes of cells are made of fat. So lipid-solube drugs like heroin, cigarettes-nictone, cocaine, they're very oily molecules. they go into the brain beautifully and that's why they tend to be addictive. Because they move preferentially into fatty tissue like the brain. Other substances which don't dissolve in fat very well, don't get into the brain very well. But there are some exceptions. Those exceptions are things that the brain needs. So sometimes if it needs a certain chemical that wouldn't be able to diffuse in very easily, it has special transporters which can scrutinise what is going past in the blood, grab goodies that it wants and move those into the brain. This is what people found when they were giving the drug L-Dopa for Parkinson's Disease. -Dop is an amino acid, dissolves in water, doesn't dissolve in brain tissue very well but it gets into the brain much betetr than it should do and the reaon is theer are these special transporters that get hold of it and shove it into the brain.
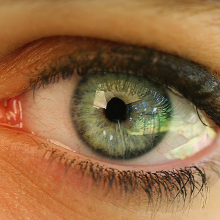
56:15 - What are floaters, and can we draw them away?
What are floaters, and can we draw them away?
We posed this question to Dr. James Johnson from Vitreous Floater Solutions in Southern California...Well, floaters are actually very common. It is an age related phenomenon for the most part especially as we get in to the 40s and older. And what it is, is a clear gel in the back of the eye starts to condense and coil less and cloud over. Hence, that what the person sees will be spots and threads and shadowy clouds or cobweb type shadows which move around in the vision. Most of these floaters are considered benign, but it is worth getting a good eye examination to make sure that it's not part of something more serious such as a retinal tear or a retinal detachment. The common advice that most people are given is to just learn to live with it and hope that it will go away. Now, the traditional treatment as I said is just to learn to live with it. There is actually a surgical intervention, a surgical procedure called the vitrectomy and it involves putting small instruments inside the eye to essentially suck out the gel in its entirety and replace it with salt water. As you can imagine, it's invasive, it has complications, commonly cataract and sometimes even retinal detachment. And as far as your reader's question about putting a coil of wire, it reminds me of a Steve Martin movie called The Jerk where he did put a little handle, a little wire on some glasses and in the movie, everybody got cross-eyed. Well, that wouldn't happen, but there's nothing you can really do to distract yourself from those floaters because they are inside the eye and they're constantly there. I have an unusual situation where I have a practice entirely devoted to treating floaters and I use a laser. So I use a highly focused laser on the floater material itself and vaporize it, convert it to a gas, the gas goes away and the floaters are gone.
Does the brain rewire around damage?
What he's referring to is a concept called plasticity and this is where nerve cells can change the connections they make from one set of nerve cells to the other set of nerve cells and in this way, there's a limited capacity of a bit of a brain that's damage so that signals can no longer go through. Then other networks or other weaker connections can be strengthened to help to bypass that blockage. There's no degradation that goes on. There's merely a strengthening, a reinforcement or a bypass via other bits of the brain to get around an obstruction or a problem which was holding things up.
- Previous What are floaters?
- Next Exploring High Altitudes
Comments
Add a comment