Cancer - Hallmarks and Hit and Run Viruses
We catch up with cancer research this week including evidence that cancers subvert stem cells to suppress the immune system, and how covert "hit and run" viral infections may be triggering a lot more tumours than we first thought. Also, joining us from the National Cancer Research Institute conference in Liverpool, cancer biologist Bob Weinberg explains how he sees cancer becoming a controllable chronic condition within just ten years. Plus, the buzz around a new tumour-spotting ultrasound technique, how a burst of electricity to the brain boosts mathematical ability, a new trick to block the brain damage done during a stroke and how bacteria store the genetic fingerprints of past viral adversaries to protect themselves in the future.
In this episode
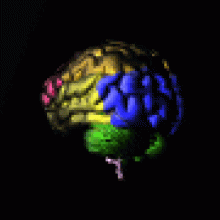
01:37 - Electrical brain boost
Electrical brain boost
A small dose of electricity to the brain can boost mathematical ability, a new study has found.
Oxford University researcher Roi Cohen Kadosh and his colleagues, writing in Current Biology, tested the effects on the mathematical prowess of 15 volunteers undergoing a process called TDCS - transcranial direct current stimulation, which involves non-invasively passing a small electrical current through the brain. The stimulus was applied to the parietal lobes (the back part of the brain), the right side of which has previously been shown to be linked to mathematical function, for 20 minutes every day over a 6 day period.
The subjects were then trained to handle a new set of nine "numbers", represented by symbols, the values of which relative to each other the subjects initially did not know. Their task was to learn them. They were assessed using standard maths testing algorithms designed to probe the subjects' understanding of how the numbers related to each other.
The results were intriguing. Subjects in which the right side of the head was connected to the positive electrode did much better on the numerical tests than subjects wired up the other way around who performed worse.
Control participants, who did not receive any real stimulus, fell somewhere between the performances of the two groups. Even more surprising, when the same subjects were re-tested months later and without having received any electrical stimulus in the meantime, those that had benefited from the stimulus initially continued to outperform the others.
According to the Oxford team, up to one person in 5 has significant difficulty handling numbers in their head. This approach, they say, could therefore make a significant contribution to boosting mathematical brain power for the numerically challenged, or just the average joe who wants to buff up his mental arithmetic!
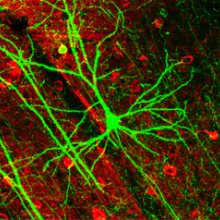
04:37 - Blocking brain damage, at a stroke
Blocking brain damage, at a stroke
Doctors may soon have a new weapon in their arsenal with which to combat strokes, which occur when the blood flow to part of the brain is interrupted.
Writing in Nature this week, UCLA-based researcher Tom Carmichael and his colleagues have found, using experimental mice, that surviving brain tissue surrounding an area affected by a stroke becomes electrically underactive. This occurs because the cells in this region increase their response to the inhibitory nerve transmitter GABA.
In the short term this is beneficial because, by suppressing the activity of these nerve cells the brain reduces their metabolic demands and therefore helps them to survive. But this state of electrical inexcitability seems to persist, which can hamper recovery in the long term. For instance, these brain regions can often take on some of the workload of the adjacent injured areas, helping to restore function.
Reasoning that re-enhancing the activity of these cells might therefore boost recovery, Carmichael and his colleagues administered an agent called L655,708, which was able to block the aberrant GABA signals in the brain.
Animals that received this treatment, which was best administered from three days after a stroke, showed dramatically improved motor function compared with untreated controls. This suggests that stroke rehabilitation in humans should perhaps also include a pharmacological component, rather than solely a physiotherapy-based regimen as is currently the status-quo.
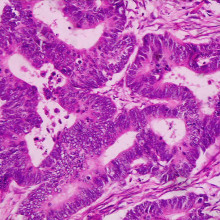
07:05 - Cancer's Own Immunosuppressants
Cancer's Own Immunosuppressants
with Professor Douglas Fearon, Cambridge University
Kat - Also in the news this week, scientists have made a breakthrough that could provide a new way to combat cancer and Cambridge University researcher Professor Doug Fearon has discovered that when cancers develop, they attract stem cells from elsewhere in the body. These stem cells, which carry a marker called FAP, seem to produce immune suppressing chemicals that prevent the immune system from attacking the tumour. But remove these cells and the cancer then becomes vulnerable to immune attack...
Doug - The problem that we wanted to solve was how did the tumour microenvironment prevent killing of tumour cells, cancer cells, by immune cells? We approached the problem by asking if there were cells in the tumour microenvironment that you actually would find in other places where we thought immune suppression would be physiologically reasonable like healing wounds or even the uterus and placenta. And it was a cell type that you could find in healing wounds, and in placenta that had first been described in 1990 by Lloyd Old, present in essentially all human adenocarcinomas. This cell could be recognised by its expression of a membrane protein which we'll call FAP, which is an acronym for Fibroblast Activation Protein. So using this as a membrane protein to identify a specific cell, we devised a genetically modified mouse strategy in which we could kill FAP expressing cells conditionally after tumours had formed in the mice, to see what would the effect be on immune killing the tumour.
Chris - So your theory is that something is stopping the immune system attacking a cancer and that these cells that appear to be recruited from around an animal's body - these ones that are FAP positive, they make these fibroblast activation protein - they're the cells you believe that are suppressing the immune system. So if you get rid of them, the immune system should be able to attack the tumour.
Doug - Yes. That was a hypothesis we wanted to test was, if we were to kill that cell and only that cell in the tumour, could the immune system then control tumour growth? The outcome of the experiment was that when we did deplete the tumour microenvironment of these cells, the immune system did indeed kill the tumour or reduce the number of viable cells in the tumour within 48 hours.
Chris - How did you get rid of the FAP positive cells in the cancers in the mice?
Doug - That's been a very nice development over the last few years, where you can target the expression of human diphtheria toxin receptor, to particular cell types genetically in modified mice, because mice don't have a diphtheria toxin receptor. And if you give diphtheria toxin, the receptor internalises that toxin, a toxin turns off protein synthesis in the cell, and the cell dies within 24 hours. So the experiment is based on the fact we made mice in which the diphtheria toxin receptor would be expressed only in FAP positive stromal cells and nowhere else.
Chris - So you put tumours into a mouse, they get these FAP positive cells in there, you delete those cells using this diphtheria toxin technique, and the tumours then regressed?
Doug - They did in 48 hours - either didn't grow or actually got slightly smaller. In addition, the number of viable cells that we found in the tumour was decreased by about a half to 2/3. So, there was, overall, maybe about 80% fewer viable cells in that tumour than in a controlled tumour, just 48 hours after the addition of diphtheria toxin.
Chris - Is this exclusive to the experiments you've done or would you find the same population of cells in any kind of cancer, and therefore, the technique would generalise to other tumour types?
Doug - Well, as I said, this cell was discovered by Lloyd Old in 1990, published in a PNAS paper, showed that it was present in essentially all human adenocarcinomas. So, if the FAP expressing cells in humans have the same function as they appear to in mice, then potentially, if we could get at that cell in humans, most human adenocarcinomas might become more susceptible to immune control.
Chris - And do you know why those cells stop the immune system attacking the tumour?
Doug - The only insight we have now, which is pretty limited, is that we know we can block the tumour killing by giving neutralising antibodies tumour necrosis factor and interferon gamma, two cytokines made by immune cells, interferon gamma, particularly by T-cells. So we presume somehow that FAP expressing cells are inhibiting the tissue response to these two cytokines.
Chris - So the next step will presumably be to ask, can you ubiquitously get rid of FAP in the long term and see what happens to these animals? Will they stay healthy?
Doug - These cells are found in bone marrow, bone, adipose tissues, skeletal muscle, retina, olfactory epithelium of the nose, tongue - so a variety of different normal tissue sites, which has not been appreciated before, and it's looking like they have some important functions in these tissues, but we're still working on that. So I don't think it'll be a therapeutic option to simply kill these cells all over the body. I think somehow, we have to interfere with their function selectively in a tumour, so we need to be a little more sophisticated. We need to know and understand what these cells do. Do they make a protein that we could inhibit in some way? Can we inhibit how they accumulate in the tumour? Those are the approaches we have to develop. We can't have a blunderbuss approach of killing them off in the entire individual.
Chris - So is that the next step then, to try and work out how you can target just those cells in just the tumour environment and not have ubiquitous killing throughout the body because there probably will be consequences.
Doug - So, what we are doing is that we've looked at the genes that are being expressed in FAP expressing cells in adipose tissues, skeletal muscle, bone and tumour. And then we go to the tumour and say, what are the genes uniquely expressed in the tumour FAP cells? Might they be related to immune suppression? Might there be targets that we could have some therapy directed at and we're right in the middle of that.

13:31 - Bacterial immune system probed
Bacterial immune system probed
Bacteria have a clever gene-based immune system that helps them fend off attack, researchers have found.
Writing in Nature, Quebec-based scientist Josiane Garneau, from Laval University, has shown that when Streptococci and other bugs come under attack from bacteria-hijacking viruses called bacteriophages or even trnamissible pieces of DNA termed plasmids, they add a piece of the genetic code from the invader to a special region of their own DNA.
The molecular equivalent of a DNA trophy cabinet, this microbial library consists of many short chunks of genetic material garnered from previously-encountered attackers. It's been termed the CRISPR region, short for clustered regularly interspersed short palindromic repeats.
This region, Garneau and her colleagues have discovered, serves as an immune memory for the bacterium. If the same bacteriophage turns up again, its DNA sequence is compared with the version lodged in the CRISPR region. If there's a match then a set of cellular scissors, directed by one of a family of genes called cas, chops up the invader's genome before it can do any damage. Understanding how this system operates could hold the key to "vaccinating" biotechnologically significant bacteria such as those used in the cheese and dairy industry to make the bugs less vulnerable to bacteriophage attack.
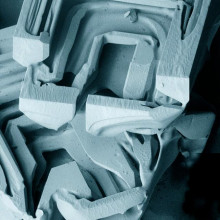
16:25 - Planet Earth Online - Supercooled Water
Planet Earth Online - Supercooled Water
with Richard Hollingham, Planet Earth Online and Ben Murray, Leeds University
Kat - Now, here's an easy science question: What temperature does water freeze at? You'll probably be forgiven for thinking the first answer that pops into your head - "it's zero degrees. Duh!" But actually, that's not necessarily the case and scientists at the University of Leeds believe that supercooled water may be implicated in the crash landing of the British Airways 777 jet at Heathrow in 2008, when both engines lost their power. Here, Planet Earth podcast presenter Richard Hollingham met Ben Murray in his lab at Leeds for demonstration.
Ben - So we just got a chilled bath. It's at minus 7 degrees C right now and in that chilled bath, I've got some bottles of bog standard mineral water.
Richard - So we can see on the top that it's minus 7 and you look in - these aren't frozen! That's wrong, isn't it? Because doesn't water freeze at zero?
Ben - Ice melts at 0 degrees C. Yes, but water does not necessarily freeze at 0 degrees C and this is quite a nice a demonstration of that. Water can exist in what's called a supercooled state, so it can exist below 0 degrees C in a liquid form. In order to turn it into ice, you have to have the right type of particle, the right surface present to catalyse the ice nucleation process or the ice formation process.
Richard - So what are you going to do to it?
Ben - We're just going to take a bottle out and as you can see, it looks like liquid water, it's moving around in the bottle.
Richard - I guess I feel that. Yes, it's definitely cold.
Ben - And now, what I'm going to do is just pour onto this glass dish. There's a little bit of ice in that glass dish which should trigger the crystallisation. Okay, there it goes. So you can now see it's...
Richard - That's amazing. It's turning to slushy ice in almost a pyramid shape as you pour it.
Ben - It fell over!
Richard - Instant ice!
Ben - So the super cold water, as soon as it hits something it can freeze on, it does so, and you can hear it. It's slushing a little bit there, I guess it's going to overflow.
Richard - So there we go. You poured a bottle of water onto a dish and as you poured it, it became ice.
Ben - Here you are. Just to prove there's nothing funny going on, we can just eat a little bit of it...
Richard - Okay. Can I try that? This is not...?
Richard - Yup.
Ben - Just cold.
Richard - It is cold. It's definitely ice. I put rather too much in my mouth then. It's quite a vivid demonstration, it's instant as well. There was no slow freezing process. It was *schwoom*! Done! You've done this many times as an experiment. You study ice and the supercooled water in clouds, but you made the connection between what we've just seen demonstrated here and the plane crash in 2008, when the Boeing 777 crash landed short of the runway of Heathrow.
Ben - I was actually made aware of this issue by a colleague in called John Morris. He has a small company down in Cambridge and he made me aware that it was something to do with ice in the fuel system that apparently caused this fuel blockage, which caused the plane to lose power as it was approaching the runway, which obviously caused it to crash land. In the crash investigation report, the official report on the accident, the engineers implicitly assumed that water droplets below 0 degrees C existed as ice. And then they went on to describe experiments they've done, where they noticed these apparent ice particles became sticky at about minus 8 degrees C. We read this report and we just thought - hang on - first of all, water doesn't freeze at 0 degrees C. Water droplets will exist and stay liquid right down to much lower temperatures. And what's probably happening in a fuel system is that water droplets are hitting surfaces and then freezing when they collide on those surfaces. So we just set about using our existing equipment that we use for atmospheric cloud research to show that water droplets, suspended in jet fuel, are actually supercooled and that's what we demonstrated. In fact, the water droplet suspended in this jet fuel only froze at about minus 36, minus 37 degrees C. So, the jet fuel doesn't catalyse the freezing process.
Richard - So in jet fuel, you assume then, with planes flying above us now, there is this supercooled water in them?
Ben - Yes. I'm obviously not an expert in aviation and jet engine fuel systems, but yes, you would expect at the low temperatures that planes fly at, that some of the water in the fuel system will exist in a supercool state, and then when it collides with surfaces, it can then freeze. I still fly, I don't think this is a major problem. It's only happened a handful of times. But it has happened a handful of times and I think is important to get to the bottom of it.
Kat - That was Ben Murray from the University of Leeds, talking to Richard Hollingham from the Planet Earth podcast.
You can see a video of this experiment at the Planet Earth Online Website here!
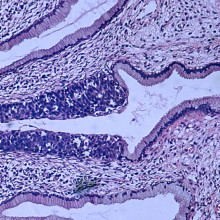
21:56 - The Hallmarks of Cancer
The Hallmarks of Cancer
with Professor Robert Weinberg, MIT
Kat - 10 years ago, a seminal paper entitled "The Hallmarks of Cancer" was published in the journal Cell, examining the state of the nation in cancer research, and making some predictions for the future. Now I'm here at the NCRI conference in Liverpool which is the UK's biggest cancer conference, bringing together scientists, clinicians, everyone in the cancer research community from around the world. And I'm really pleased to be joined by one of the authors of that report. The two authors were Douglas Hanahan and Robert Weinberg, and here's Robert Weinberg with me today, and he's going to be talking at the conference about where we are 10 years on from the paper, so good afternoon. Hello.
Robert - Thank you for having me here. It's my pleasure.
Kat - That's great. Let's talk a little bit about your original paper, "The Hallmarks of Cancer." So, what did you and Doug actually look at in that paper?
Robert - Well Doug Hanahan and I are scientists who are interested in understanding what causes cancer, how normal cells can become converted or transformed into cancer cells. One day, we were at a conference together in Hawaii, walking down the mouth of an extinct volcano, and we came to the notion that it might be very useful if we were to codify the principles by which normal cells become converted into cancer cells. The cancer research literature was vast, but we believe there was a small number of underlying principles by which we could explain the entirety of the cancer formation process. And Doug Hanahan came up with the title, "The Hallmarks of Cancer." There were six of them in fact.
Kat - So what are these six fundamental principles of cancer cells?
Robert - That cancer cells stimulate their own growth; that they're resistant to inhibitory signals that might otherwise stop their growth; that they can proliferate, they can multiply forever; that they can engender the growth of blood vessels into tumours, to supply nutrients to the tumours; that they can become resistant to their own programmed cell death - sometimes called apoptosis; and that with the passage of time, they can become invasive and metastatic. That is to say, they can spread throughout the body of the cancer patient.
Kat - When you presented this gathering of ideas to the scientific community in your paper, how well was it responded to?
Robert - Well, like most review articles, this one was such a review article, we expected it to be cited briefly and then disappear like a stone, thrown into a small pond, but in fact, it was cited more and more in the years since 2000. I believe, even 10s of thousands times much to our astonishment. We never expected this.
Kat - It seems a bit strange to think that no one had actually sat down and thought about what is cancer actually like before that point.
Robert - Well, many people had thought about what cancer is like from many different perspectives, but we thought there might be a common perspective and a common set of rules by which cancer cells arise, and those are the rules we articulated. Now, 10 years later, we're doing an update on that and we're almost done writing that update on "The Hallmarks of Cancer" to begin to indicate what new insights have happened since then.
Kat - And so, where are we now? What's happened over the past 10 years? Did you actually make any predictions back then? Have they come true?
Robert - Well actually, we didn't make that many predictions but we have learned a lot in the past decade. Cancer research has thrived and we learn more and more about why cancer cells proliferate uncontrollably. One thing we've learned is that cancer cells must somehow evade destruction by the immune system. Another thing we learned is that cancers or tumours are complex tissues, and indeed contain as many distinct cell types as normal tissues do. And the various distinct cell types of the tumour interact one with the other, in order to create this ever growing tissue which we call a tumour.
Kat - We heard earlier that fantastic new research from Doug Fearon and his colleagues and it's really looking at the role of cancer, almost being like being a rogue organ. So you've not just got the cancer cells, but you've got all the supporting cells that have been hijacked into this tumour.
Robert - That encapsulates the finding precisely so. Still, we would like to be able in the future to mobilise the powers of the immune system in order to eliminate tumours, and that's not a place where we're at yet, even though people have been trying for two decades to direct the powers of the immune system to eliminate tumours that have arisen in the body of the cancer patient.
Kat - You're now revisiting your work. You're writing a new paper, "Hallmarks of Cancer 2: The Revenge" or something. Have you thought of a name?
Robert - I think it's just going to be, "Hallmarks Revisited." Nothing more dramatic and we're not going to make a follow up movie either.
Kat - And where do you think we're going to be going in the next 10 years? Will we be curing many more cancers and how is this research leading to that?
Robert - Well there's two areas of this research: One, what causes the cancer and two, can you use the findings on the causes of cancer, in order to develop new types of therapies. And in fact, over the last 30 to 40 years, we've learned an enormous amount about why cancer cells grow uncontrollably. If you would've asked me about that question three or four decades ago, I would've shrugged my shoulders and told you I have no idea. But now, we understand that in enormous detail, but unfortunately, only a small number of those insights have been converted into truly useful therapeutics. Over the next decade, we're going to see an explosion of developments in new types of drugs that are created which can act either on their own or more often, in concert with other drugs, to really wipe out tumours, or at least hold them at a small or slow state of growth that will be tolerable for the cancer patient.
Kat - So this is the idea of much more targeted therapy that's aimed at the molecular faults in a tumour, rather than just, "Oh, it's that kind of cancer."
Robert - Precisely so. Many of the chemotherapeutics we use today were invented three and four decades ago, and are simply toxins that happen to kill cancer cells slightly more easily or readily than they kill normal tissue, but of course, they create a lot of side damage, side effects. In the future now, we will increasingly be able to tailor-make drugs that specifically hit the cancer cells and thus the tumour, without creating damage, without wreaking havoc in adjacent normal tissue.
Kat - And we've made a lot of progress in treating cancer over the past few decades if you look at the change in survival statistics, maybe even just over the past 20 or 30 years...
Robert - Yes, indeed.
Kat - ...many more people do survive cancer and live for much longer, but obviously, we have a lot further to go. Where would you like to see the state of cancer treatment and survival in 10 years?
Robert - Well, I would like to see solid tumours being treated more effectively. Right now, advanced solid tumours from the colon and the pancreas, and the lungs are really formidable enemies, and we don't really know how to stop them. It would be nice - I think it's even realistic to assume that 10 years from now, some of those tumours will be stopped in their tracks, caused to shrink. They may not be caused to totally disappear, but they will be kept at a small size that will render the patient fully normal in terms of his or her lifestyle, and will create a chronic - albeit, tolerable disease. That's a reasonable prospect 10 years from now, without promising any major miracles.
Kat - Now that's something absolutely fantastic to look forward to. That's Robert Weinberg from MIT.
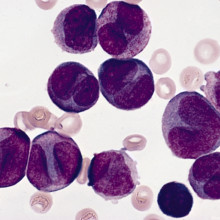
I heard that radiation treatment for breast cancer can cause leukaemia. Is that true?
Chris - Yes, unfortunately it is true because the way in which some cancers are treated is with radiotherapy. Radiotherapy is a form of ionising radiation like x-rays, and as a result, when that ionising radiation goes through your body, it does damage cancer cells, and it tends to damage cancer cells more than healthy tissue because cancer cells are more vulnerable to damage because they don't have such good DNA repair mechanisms. But the point is, it can still damage healthy tissue.
And so, in the course of dealing with one tumour, it can increase the risk - it doesn't necessarily give you cancer - but it can increase the risk of developing another type of cancer. Very often, bone can be targeted or bone marrow cells, and therefore, you can get secondary tumours developing later in life as a consequence of having been treated.
But if you take certain chemotherapy drugs, they don't necessarily have that same risk. They're not ionising radiation, so it depends on the kind of treatment that you get.
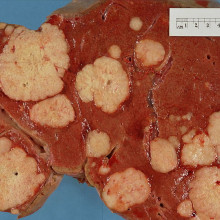
Is metastasis a behaviour?
Chris - Indeed it is, because what's happening when a cancer spreads is that the cancer is spitting off cells that have gained the ability to autonomously migrate through other tissue layers, ignoring normal boundaries, to move to a distant part of the body. They then move into that part of the body where other cells, which are not cancerous but are healthy tissue, have been manipulated by chemicals produced by the cancer to flock to that area, and produce - for all intents and purposes - a nest for that cancer cell to move in to, and then begin to grow. Then you develop another secondary cancer and that's the metastasis. It's a complicated process that scientists are only just beginning to understand.
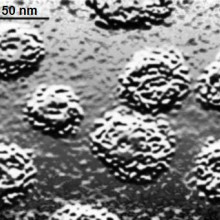
31:13 - Hit and Run Viruses Leave Tumours in their Wake
Hit and Run Viruses Leave Tumours in their Wake
with Dr Stacey Efstathiou, Cambridge University
Chris - Now for many years, scientists have known that viruses are the direct causes of some cancers and probably the best described example is HPV, that's the Human Papillomavirus which is linked to cervical cancer. But recently, researchers at Cambridge University uncovered evidence that viruses might be involved in the causation of a much wider range of cancers than we first thought. One of the scientists behind that discovery is Dr. Stacey Efstathiou. He's with us now. Hello, Stacey.
Stacey - Hi, Chris.
Chris - Firstly Stacey, how do scientists actually think that viruses cause cancer in the first place?
Stacey - Well, there are a number of different ways that viruses can cause cancer. From a historical perspective, if we look back maybe 100 years ago, it was first shown that if you took tumours from chickens - sarcomas - you could mash them up and take a filtrate which lacked anyof these cells, inject it to a normal chicken, and it would develop a tumour. And it was subsequently shown that the virus responsible is a retrovirus and retroviruses are special viruses, that as part of their life cycle, they integrate into the host genetic material. In that particular case, the virus encoded its own oncogene which had captured historically from a cellular oncogene...
Chris - An oncogene, being a gene that make cells grow, causing cancers.
Stacey - Causing proliferation of the cell. So, that's one example that viruses causing cancer, the capturing of an oncogene and subsequent expression in a cell.
Chris - And they knew from that experiment that it had to be a virus because if you mashed up the cells to destroy the cells then the only thing that could survive that process presumably is a virus, otherwise it's too small.
Stacey - Absolutely. So that was a landmark discovery and that it was filterable agent, a very small entity, a virus which actually transmitted the tumour from chicken to chicken.
Chris - What's the benefit to a virus of transforming, making a cell become malignant in the first place?
Stacey - Well, I guess you'd say that there isn't much of a benefit. I mean, these are accidents that happen when a virus can't complete its normal lytic cycle, and as by-product of that, a tumour occurs. So, many viruses encode genes which will push the cells into replication and cause those to proliferate, to help the virus be produced. But normally, the outcome of that is death of the cell. It's only in specific circumstances where you haven't got complete lytic destruction of a cell by a virus that a tumour can arise.
Chris - So in other words, the viruses are making things that make cells grow more because the cell that's growing more is good for a virus to grow in because growing cells are more metabolically active.
Stacey - Absolutely.
Chris - But occasionally, an accident happens and something goes wrong genetically which damages that cell's DNA or re-arranges it in some way, so it can become potentially malignant.
Stacey - Absolutely and a classic example of that - you mentioned already - is Human Papillomavirus which causes cervical cancer. It's a real rare event where one gets an integration of and papillomavirus which is not a normal part of the viral life cycle and when integration happens, you can get expression for one or two of the viral genes which cause cells to proliferate. But because the virus is integrated, it can't complete its longer life cycle and the consequence is proliferation and tumour formation.
Chris - Integration being where the virus has put its genetic material inside the cell's genome.
Stacey - Absolutely, that's right.
Chris - So that's all very well. We can go looking at tissues and we can look for the viral hallmark in the cell's DNA. You've done some work now saying that actually, if we just go looking for cells that bear a viral hallmark of having been damaged in this way, you found some, but actually, there's evidence that viruses may be underlying a lot more in the way of cancers than we first thought.
Stacey - Yes, sure. I mean, this has been a longstanding issue and in the field of virology, the so-called 'hit and run' hypothesis of a tumour formation where a virus can enter a cell, express some genes, make a change to a cell, to cause it to become cancerous, but then disappear. Actually, be lost without trace from a tumour. But of course, how do you study that? It's a very difficult question to address. And so, what we tried to do is to experimentally establish a system where we could examine whether a virus could actually undertake a hit, i.e. enter a cell and expresses something, but then be cleared, once the tumour had been initiated.
Chris - But obviously leaving a bit of a stink behind in the form of the genetic damage you were talking about that could make that cell cancerous.
Stacey - Absolutely.
Chris - And if you went looking, you'd say there's no virus there, but the footprint is there nonetheless.
Stacey - Exactly, so if the hit and run hypothesis is correct of course, it may be that viruses could actually be associated with many more cancers that we currently appreciate because there's no means of associating that virus genetically with the particular tumour.
Chris - How did you do it?
Stacey - Right. So this was actually quite a tricky experimental procedure in which we use transgenic mice which had copies of an activated oncogene, but would only be activated once a virus, which expresses a particular enzyme, which we had engineered into the virus, was expressed in that cell to mediate activation of a cellular oncogene. So, once we infected the mice, any cell that got hit by the virus when you got expression of this enzyme will then trigger this oncogene, that will induce a tumour. And so, the question was, if we follow that tumour, was the virus always retained in that cell or was there subsequent loss of the virus? And what we found was that if we looked at the tumours from animals that inevitably, the viral DNA had been lost from the tumours but the tumours brought all the hallmarks of a virus having once been there. We took this study on further by showing that one could actually vaccinate these animals prior to delivery of this viral enzyme that we cloned into the virus, and show 100% protection against the virus induced tumours. So, this was an experimental system, demonstrating hit and run oncogenesis, and showing that vaccination would be very effective in preventing tumour induction.
Chris - And just briefly, what do you think the implications are of this? This suggests that a lot of the cancers that we're seeing are caused by other things, there may actually have been the early evolution of that cancer, being a virus there, causing this problem.
Stacey - Sure and so, if one looks at certain cancers where a virus is sometimes associated but not always associated with the cancer, could it be that in those cancers where the virus has been lost that there have been an initiating event at one time in the past? And of course, the only way one can realistically prove this in the human population is to develop novel vaccines, so I think the impetus now is to put a lot more effort into the development of vaccines and to roll them out to see if we can actually prevent tumours which you wouldn't necessarily suspect of having a viral aetiology.
Chris - Stacey, thank you. That's Dr. Stacey Efstathiou. He's from the department of pathology at the University of Cambridge
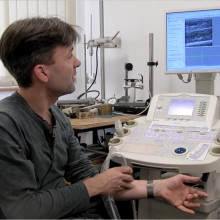
38:53 - Naked Engineering - Ultrasound
Naked Engineering - Ultrasound
with Dr Graham Treece, Cambridge University
Chris - Now, it's time to join Meera and Dave, and they've gone to a noisy car park to explore the engineering basis of ultrasound. Something of course, which is very useful in the diagnosis of, and screening for cancer.
Meera - This week on Naked Engineering, Dave and I are going to be looking into how ultrasound works. Now Dave, you do always take me to glamorous locations. We're lingering on the street, outside of a multi-storey car park. Now what has this got to do with ultrasound?
Dave - Well, to understand how ultrasound works, it's easy to start off with conventional sound. Sound is basically a vibration in the air which travels through the air. So when I'm talking here basically, I'm making the air vibrate in my throat, that's causing the air in front of it to vibrate, the air in front of that to vibrate, until it makes your ear vibrate, so you can hear me. Now, what's interesting and the reason why I brought you to this beautiful location is if I make a very loud noise, about 40 metres away, there's a great big wall. And so, if I make that loud noise, there's going to be large amounts of sound vibration going out to that wall. It's going to hit that wall. It can't carry on. All it can do is bounce back and we should get an echo.
Meera - Okay, so we've got a lot of cars around us though, so I guess the noise you make is going to have to be quite loud.
Dave - Yeah, you're right. It could work with a clap. The problem is, my hands now hurt after clapping far too much and so, I'm going to try and make a very loud beepy noise.
Meera - Okay. Let's hear the echo then.
(Beep sound)
Meera - Yeah. I really heard that echo actually and it only took about half a second to come back.
Dave - Something like that, yes. Sound travels at 300 meters a second, so it's got to travel there and come back again, and so, probably half or a third of a second. If you get a bit closer, it should be a bit quicker. So, if you hear a whole series of echoes of a whole series of objects, you work out how far away they are by how long it takes the echo to get back.
Meera - Is this the principle used to try and image things or see where something is using sound?
Dave - That's right. If you know the direction the sound has gone out in and come back in, and how long it took, you know exactly where it is. So you can build up a 3-dimensional image. It's also how sonar works, so how you find submarines underwater.
Meera - Another form of sound that can be used for imaging is ultrasound and somebody that uses this for medical imaging is Graham Treece, based at the engineering department here at the University of Cambridge...
---
Graham - Ultrasound is a higher frequency than normal sound, so it's not stuff that you can actually hear with your ears, but it is still a vibration. It's just at a much higher frequency. High frequency sound actually travels into skin. It won't travel past bone, but it travels in soft tissue. So, using sound, you can see into anything which is soft tissue so that includes imaging babies in the womb. That's what most people have heard of, but includes imaging say, liver or kidneys or muscles, or anything which is soft tissue really.
Dave - I guess, normal sound would do the same thing, but it's got really long wavelengths, so you wouldn't be able to see any good detail.
Graham - You're quite right. The wavelength is much longer and that means that the resolution with which you can see is no good for actually looking at the detail inside people. Yes, that's right.
Meera - And so, you've got a setup here of an ultrasound machine. How does this all work together to provide an image?
Graham - Most ultrasound probes are a 1D array of little crystals, say, 200 odd crystals in a row. Those crystals, when you fire them with a little bit of electricity, vibrate and that vibration generates the ultrasound. The frequency with which you used the electricity to fire them generates a particular frequency of ultrasound.
Meera - You usually have to apply a gel onto the skin before actually using this probe. Why is that necessary to help image?
Graham - What you're seeing in the image is the amount of sound reflected back from tissue, and sound reflects when there's a big change. So for instance, if you clap or ping next to a wall, the sound reflects off the wall because the air is not very dense at all and the wall is very dense, so you get the sound reflecting back. It's the same in tissue. You're seeing reflections of, mainly, different densities in the tissue. Now the problem is, if you just hold a normal ultrasound probe in air, the air is not dense at all, so all the sound basically just reflects straight back without ever going into the body at all. So you have to have what's called a coupling gel - or in fact, water does a reasonably good job as well - between the probe and the tissue to remove all the air so that the sound actually gets into the tissue, rather than just coming straight back at soon as it hits air.
Dave - So the probe is producing a beam of sound into your body and it steers that around and listens for reflections coming back.
Graham - That's actually quite an important point. Yes, of course. If you only had one element producing sound, it will produce sound in all directions and you wouldn't know where the reflections were coming back from. And there's actually a little lens on the surface on the probe which focuses the sound in a plane. So effectively, you get a sort of thin beam of sound coming out.
Meera - Okay, well you do have some of the gel and also some water next to you. So could we perhaps see this in action? There's no chance of you being pregnant, so I guess you're just going to be putting it on your arm?
Graham - Yes, that's right. In fact, the probe I'm using is a higher frequency probe. You'd use a lower frequency probe for pregnant women because the lower frequencies see further into the body and higher frequencies don't see as far, but with better resolution.
Meera - Okay, so you've just dipped the probe into some water and it's now on your arm, just below your wrist, and an image has come up. It's actually quite clear. It's a grayscale image, but you can quite clearly see the muscles.
Graham - Yeah. You can see all the tendons and the muscles in the image. In fact, if I wiggle my fingers, you can see different bits of my muscles moving as they're wiggled. What's happening is that little pulses of sound are being sent into the tissue and then they're received back. The next element along in the transducer is then fired and that sends a pulse into the tissue and that's received back, and that's repeated lots of times, so you have 1 to 200 different echoes and responses. Now each of those is a vertical line in the image that you're seeing. And so, when you put them all together, you get what looks like essentially a 2D cross section through my muscles.
Meera - This explains just how the process works to allow us to see inside our bodies. But you're using ultrasound in a slightly different way in your research, Graham...
Graham - Normally, with ultrasound images, you see just the amount of sound that's being reflected back from tissue boundaries, and that's very good for looking at lots of things. But what we're looking at is using the same signal, so the same ultrasound, but to look at tissue stiffness. This is really interesting because there are quite a lot of physiological conditions where stiffer tissue means something. So for instance, if you have a lump or a bump, in a sense, we're trying to produce a way of imaging that lump, a bit like manual palpation. You know, clinicians prod a lot and what they're feeling for is essentially stiff bits under the surface. Well if you can image them instead, you can see how stiff they are and exactly what shape they are, and how deep they are, and you can get it with a much better resolution than just feeling with your fingers.
Dave - And I guess, if you're using an ultrasound probe, you can go a lot deeper than you can do with poking your fingers.
Graham - Yeah. In general, that's true. So, with this technique, you can see a stiff thing which is only say, a millimetre across, 4 or 5 centimetres deep and you probably wouldn't feel that if you prodded.
Meera - How does this actually work then? So why are you able to see differences in stiffness, and to what scale?
Graham - In normal ultrasound, what you actually see on the image is the amount of sound being reflected, as I've said. Now, we can use exactly the same signal, but what we do is acquire lots of ultrasound images and then we compare them, and by looking at the way that the tissue moves between each of these images, we can work out how it's deforming as you press slightly with the probe. And that can give us information about how stiff the tissue is. So, if you imagine a very stiff thing embedded in some very soft material, if you pushed it, what would happen is the soft material would squish together, and the stiff bit would just move without squishing, and that's what we're detecting. Essentially, we're measuring the deformation and then you take the gradient of that, which gives you something called strain, and we can relate that to the stiffness of the tissue.
Meera - What makes it better or why is it beneficial to use this over current methods of looking for, or finding, tumours?
Graham - Well you're seeing something completely different. So, normal ultrasound is quite good at looking at soft tissue tumours, but it doesn't show you stiffness. So, there are already reasons to believe that this will improve either diagnosis of tumours, so we have a pilot study looking at breast tumours at the moment, or at least help to decide whether to go ahead and have a biopsy which is what normally happens if someone is suspected of having a tumour. So it may help, for instance, in reducing the number of biopsies that need to happen.
Meera - Okay, well I look forward to seeing these in our hospitals soon. Thank you very much, Graham and thank you, Dave. That's it for Naked Engineering this week.
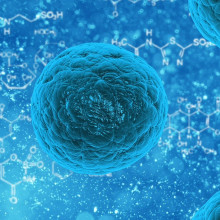
Do research cell lines mutate?
We put this question to Professor Robert Weinberg:
Robert - The more we learn about cancer cells that are grown in the petri dish, outside of the body of a mouse or human, the more we appreciate that with the passage of time, they become less and less representative of the cancer cells that are in the body of a cancer patient. As a consequence, one has to devise various kinds of new cancer cell lines that more closely approximate the cancer cells inside the body of a patient.
Kat - And do we have to have more effective animal models that better reflect real tumours?
Robert - Yes, indeed. Ultimately, the most effective way of modelling cancer is to trigger a tumour that arises spontaneously within a mouse for example and studying the way that tumour develops and grows within the mouse, that much more closely recapitulates the growth of tumour, than does for example, a human cancer cell line that is implanted in a mouse and grows and forms a tumour there.

Are viruses intelligent lifeforms?
We put this question to Dr Stacey Efstathiou:
Stacey - Well as a virologist, I'd say yes, very intelligent! I mean, they're elegant life entities that can reproduce themselves, that can cause persistent infections, reactivate. A different type of intelligence, no brain cells involved.
Chris - Indeed, but it depends whether you believe a virus is alive or not. I mean, my own belief is a virus is not alive, it's just an infectious bag of genes.
Stacey - It is an infectious bag of genes and it undergoes evolution, so in some ways, you could say it is a life form.
Chris - But not intelligently designed, I hasten to add...

What would the world be like if we didn't have viruses?
We put this question to Dr Stacey Efstathiou:
Stacey - We wouldn't have colds and flus, and I guess it could be a really nice place, but of course we've evolved with viruses, over millions of years. Our whole immune system has been evolved to respond to that infectious assault. So, if we didn't have viruses, our immune systems will be very different and what will the consequences of that be? I'm not sure, but maybe it wouldn't be very nice.
Chris - I think they would evolve anyway, to be honest.
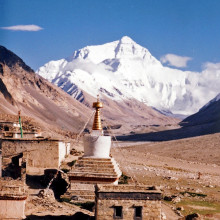
50:29 - Does a mountain make the day longer?
Does a mountain make the day longer?
We put this to Dominic Ford from the department of physics in Cambridge:
Dominic - There are a couple of interesting differences in what you would see around sunrise and sunset on top of a tall mountain. First of all, there would be difference in how long the sun would spend above the horizon. You can think about that by imagining that the Earth is a ball and if you're standing on the surface of that ball, it appears like a flat plane. It covers exactly half of everything you can see. Whereas if you move away from that ball up a tall mountain, it starts to recede away from you and appear like a globe in the sky.
Now, the Himalayas aren't actually very tall in comparison to the radius of the Earth. Everest is about 9 kilometres high and the earth is 6,000 kilometres in radius. So that means that even at the top of Everest, this ball would only appear about 174 degrees across as compared to 180. So that would make a difference of about 10 minutes in sunrise and sunset times, if you assume that the Earth is a perfect sphere and Everest is the only mountain on the surface of the Earth.
Now in fact, that's not quite right because obviously, Everest is in the middle of the Himalayas, and you'll be familiar with the fact that if there's a tall hill on the horizon, then the Sun will set behind the hill somewhat before published sunrise and sunset times.
Diana - So on the top of Everest, the Sun wouldn't spend that much more time over the horizon because it would set behind the mountains, but what about the bit in between sunset and total darkness?
Dominic - Now, there's a slightly different answer because when we talk about hours of daylight, we tend to be talking about when we perceive it gets light, and when we perceive it gets dark, rather than when the Sun is rising and setting. That's to do with twilight times and how long the sky appears bright after the Sun has set. In the UK, we're quite used to having a lot of twilight and that's to do with the fact that we're at a high European latitude and when the Sun sets, it sets at an oblique angle, and it actually spends quite a long time sitting on the horizon as it's setting.
Everest of course is close to the equator and at equatorial latitudes, the sun sets more or less vertically downwards. So it sets very quickly, and you don't have much twilight time as the Sun is setting.
Moreover, twilight is caused by the scattering of sunlight off particles in the Earth's atmosphere and at the top of Everest, you're above most of the Earth's atmosphere. So, you have a lot less light being scattered and twilight will appear a lot darker. So once the sun has set, it will get dark relatively quickly compared to what we're used to. So you might find actually, there's slightly less daylight time on top of Everest than you would see certainly here in the UK.
Comments
Add a comment