Earthquakes: Science on Shaky Ground
This week, 10 years on from the earthquake that caused the Fukushima disaster, we're looking at the science of earthquakes, new techniques to protect ancient buildings from quake damage, and are we any closer to predicting these awesome forces of nature? Plus in the news: Why countries across the EU stopped, and then restarted, using the AstraZeneca vaccine, how lightning helped life on the early Earth, and do we owe our fine sense of touch to our fingerprints?
In this episode
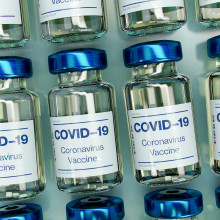
01:03 - Concerns over the AstraZeneca vaccine
Concerns over the AstraZeneca vaccine
David Spiegelhalter, Cambridge University
A suspected link to the development of a rare syndrome involving the formation of blood clots in the brain's blood vessels led to 18 European countries temporarily halting their use of AstraZeneca's COVID-19 vaccine pending an investigation by the EMA, the European Medicines Agency. The EMA itself though, while it investigates, is urging EU nations to continue to use the vaccine, which has so far been administered to over 17 million people, owing to the very real threat posed by massive surges in COVID-19 cases across Europe. That said, some countries, like Belgium and the UK, elected not to interrupt their vaccine rollouts. In the end, it all comes down to balancing risk: the risk from a very rare, and possibly unconnected medical condition, versus the relatively much bigger risk of succumbing to COVID-19 infection and its other sequelae. Chris Smith spoke to Cambridge University's David Spiegelhalter Professor on the Public Understanding of Risk, to get a handle on what's going on...
David - I think it’s quite complicated, because the story as it was last week, was about blood clots, like deep vein thrombosis and other things. And I looked at that data, what was being reported by AstraZeneca in terms of the number of adverse reactions. They didn’t look like anything special at all, but it’s since come out that real concern is, from both Norway and Germany in particular, is a particular special form of thromboses, known as cerebral sinus venous thrombosis, and it’s associated as well with low platelets. So it’s clearly quite a rare syndrome, and in Germany in particular, they’ve had 1.6 million vaccines, and have had 6 or 7 cases of something like this, and it tends to be in younger women, and some have died. And so I can understand that there is a concern about the AstraZeneca vaccine.
Chris - But policy makers have to make decisions all the time, don’t they, about what you’re going to do and what the cost might be? And what you’re not going to do, and what the cost might be, and it is really important not to chuck the baby out with the biological bathwater, because by deferring vaccination while you investigate a very remote risk of something happening, you end up costing people the chance to be vaccinated in the interim. One commentator in France pointed out that for every 100,000 people you don’t vaccinate over the age of 50 for one day, that will translate into 5 deaths, I haven’t checked that statistic, but it does sound plausible.
David - Certainly, any delay will translate into deaths, especially as the continent has seen an increase in cases every day. And so, this is a really precarious situation they're in with a very low vaccination rate. And in that context, the cautious approach that the regulatory agencies are taking by suspending the vaccine while they investigate, quite reasonably, doesn't seem to me and many others, that this is the cautious approach. Actually, the default should be to try to get as many jabs as possible into people's arms, knowing that will save lives, rather than stopping the roll out, possibly decreasing even more vaccine acceptance by the population, who are already slightly skeptical because of the possible small risk of an adverse effect.
Chris - So statistically speaking, how should they investigate something like this, you're advocating that they should carry on business as usual, but behind the scenes, obviously be taking this seriously. That's how we have a healthy pharmaceutical industry, let's face it, but what should they be doing in order to work out whether or not there is a genuine effect?
David - This is what the European medicines agencies will be doing at the moment, they'll be collecting data on adverse events, which are reported, publicly reported, in the UK. And, because we've had three of those particular conditions under the AstraZeneca vaccine, one under Pfizer so far. So they'll be collecting those and looking at them, you know, whether they cluster by a particular age, or sex of people, but also comparing it with how many we would have expected anyway. That's the crucial thing, nasty things happen. People do get blood clots. And so how many would we expect anyway, and is what we're observing way above what we would normally expect to happen just by bad luck.
Chris - But as Stephen Evans, who's professor of biometry at the London school of hygiene and tropical medicine has pointed out, if you look at people with COVID, 30% of the time, they have changes to their clotting.
David - Well, I mean, that's the whole issue, cost and benefits of the two actions. Certainly by not vaccinating people, you are exposing them to considerable harm, including the risk of blood clots.
Chris - You raised an important and interesting point when you said there's also Pfizer's vaccine, and we've had cases of this there. Are they broadly comparable in terms of when you see these side effects, are we seeing roughly the same number of these adverse events in both vaccines? Therefore, again, arguing that this is not a vaccine specific effect, it's perhaps just a consequence of having a vaccine.
David - They haven't got enough data to show that, but one of the concerns there's been is about low platelets thrombocytopenia, that's been observed or linked to the Pfizer and the Moderna vaccine as well in the States. Again, the causal link hasn't been proved, but we still can't say whether this is a vaccine effect, I think, or a product effect. This is what EMA will be looking at very carefully, indeed.
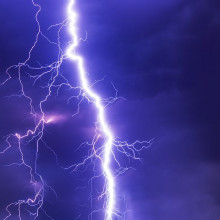
08:11 - Lightning's role in the beginning of life
Lightning's role in the beginning of life
Benjamin Hess, Wheaton University
To Earth science now and the origins of life on our planet; and when Benjamin Hess was a student at Wheaton University in Illinois, he had no idea that a local storm would change the course of his career and produce a remarkable piece of geology that has shed light, or rather, lightning, on how life may have got started here. In a new study, which he explains to Phil Sansom, he’s shown how bolts of lightning may have first endowed the early Earth with one of the crucial building blocks of life: the element phosphorus...
Benjamin - Basically lightning struck somebody's back garden, their property nearby. They called up the department of geology at Wheaton College and were like, "Hey, there's this thing in our backyard, do you want to come check it out?" And some professors showed up and realised it was a lightning strike.
Phil - And when you say "it", what exactly was it?
Benjamin - It was a lightning strike glass called a fulgurite. So when lightning hits the ground, it heats it to thousands of degrees Celsius and melts it immediately. And then it reforms. So it was like this tree trunk-like structure under the ground, with branches coming off the bottom.
Phil - And the size of a tree trunk too, yeah?
Benjamin - Yeah. So the total mass that was collected was about 25 kilograms of material. So like a fair sized tree trunk under the ground basically.
Phil - Is that kind of geologist Christmas?
Benjamin - Yeah. I mean, it's rare to find something so interesting in the flat plains of the Midwest. Let me tell you that. And I just started prodding at it basically, with whatever analytical techniques I had access to at Wheaton College. And there were a few little small spheres of something, that I didn't know what they were, using the scanning electron microscope, which essentially tells you the elemental composition and the structures of the things you're looking at, that actually allowed us to finally figure out what these metal spherules were. And it turns out that they were an iron phosphide, and phosphide is a reduced form of phosphorus, meaning it's no longer bonded to oxygen, which is really weird because we live in a very oxygenated atmosphere. So it's hard to make that happen.
Phil - So a rare mineral?
Benjamin - Yeah. Something that you really would not see. I've never seen a phosphide before in any rock. When I first found it, I was kind of like, huh, that's strange. But when I started reading the literature on what this mineral is, it's a mineral called schreibersite. The most well-known locality where it shows up is in meteorites, because there's less oxygen out in space when meteorites are forming. And it turns out schreibersite is thought to be one of the most widespread sources for phosphorus for life on early Earth, the formation of life.
Phil - Wow. This is a big jump.
Benjamin - Yeah, that's what I thought too. I was like, this is crazy. What's this meteorite mineral doing in this lightning strike glass, and you know, meteorites and origins of life. And it's like, hold on. This could actually be a really big story.
Phil - How so? Where is phosphorus important to the picture and why is it such a big deal to try and get a hold of it?
Benjamin - Phosphorus is one of the essential elements for life. It forms a lot of structural and functional elements in cells. Like it makes up the backbone of DNA and RNA, the double helix structure. The problem is it's trapped in minerals that are common, but insoluble and unreactive. So you basically can't use the phosphorus. So the question is where do you get phosphorus, that's free to react and make molecules needed for life.
Phil - And it's this mineral?
Benjamin - Yeah, that's one of the best solutions scientists have come up with. They found this phosphide mineral, schreibersite, because it readily reacts with water to free up phosphorus. But we saw this in a lightning strike and we thought, wait a minute, maybe lightning could also be a source.
Phil - As opposed to meteors, you mean?
Benjamin - Not as opposed, but in addition.
Phil - How do you actually figure out then, whether this is a likely thing to have happened billions of years ago?
Benjamin - That is really the big question that took up the bulk of the time writing this study, trying to come up with a convincing estimate. The three big things are, you need to know how many lightning strikes are happening. You need to know how much phosphorus was in the rocks that they were striking, and you needed to know how much phosphorus each lightning strike turns into schreibersite or something similar that can be available for prebiotic chemistry. Based on our calculations, we think that lightning was providing about as much phosphorus as meteorites.
Phil - Doesn't it kind of remind you of a Frankenstein sort of situation? I mean, the popular idea of creating life involves a lightning strike. And it's crazy to imagine that this was partly what actually happened for us to come along.
Benjamin - Yeah, absolutely. And the cool thing about this mechanism is it can operate on other planets as well. After the solar system forms meteorites kind of get cleared out by planets. So there's kind of a cutoff window for when they can provide enough phosphorus for life. But if you have a stable atmosphere, lightning is a mechanism that can operate indefinitely.
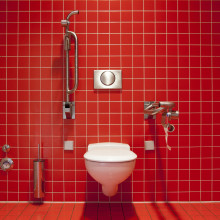
14:22 - Finding COVID variants in sewage
Finding COVID variants in sewage
Javier Martin, National Institute for Biological Standards and Control
As we emerge from lockdown, keeping tabs on where COVID-19 might be about to rear its ugly head again, as well as watching for the appearance of viral variants that might be able to sidestep our vaccines, is going to be key to keeping the country moving. And most scientists agree that mass testing in one form or another is crucial. But how do we do this in a way that's not inconvenient, onerous and costly? Well, researchers at the UK's National Institute for Biological Standards and Control, have found that they can use...what we put down the drain...as a sensitive way to track the activity of COVID in the population and also spot the early emergence of variants we're worried about. It stemmed from an existing project to use sewage to screen for polio; Chris Smith spoke with Javier Martin, one of the brains behind it...
Javier - There was early evidence that there was high concentration of the virus genetic material in stool samples from infected people. So since we have been carrying out surveillance for polio virus in wastewater samples, we thought that we could adapt our techniques to detect the virus causing COVID.
Chris - So you were already doing this for polio?
Javier - Yes. For many years now, yes.
Chris - Does this involve, then - not to put too fine a point on it - just going into the sewage farm and saying, "can I have a sample of what's coming down the sewer pipe, please?"
Javier - We were lucky because we've been doing this for many years now, so we regular samples supplied by the sewage plant every month. So we had samples from before and during the pandemic to look at.
Chris - Presumably, then, this bottle arrives, which is wastewater from different parts of the country; how do you process it?
Javier - Yes - you clear it up from any solids that might be there, by using filters to concentrate particles such as viruses.
Chris -
What, is it whole viruses that you're collecting, or will there just be bits of genetic information from these viruses in there?
Javier - We are not sure what if they are viable virus. We think they are not infectious, they're probably particles that are somehow damaged, but they contain genetic material that's sufficient to detect it.
Chris - And you can get that genetic material out and read it, can you?
Javier - Yes.
Chris - And can you detect things like variants doing this? If you can read genetic code, does that mean you can basically spot a variant, or spot the rate of change, how a variant is increasing its population as the infection spreads through the country, or a variant emerges, for example?
Javier - Yes. We're trying to quantify the amount of genetic material in wastewater; also sequencing so we can identify those mutations that correspond to variants, and therefore monitor them.
Chris - What's the point of doing this? Why do you think this would be useful?
Javier - I think it would be useful to help understand how these viruses meet between people, and also to possibly establish early alert systems for such variants becoming predominant in a population. Also, what you find as water is obviously what people are excreting, so you are not biased by the sampling of clinical samples; and they represent also asymptomatic infection, so a more detailed picture of what's happening.
Chris - I suppose one constraint, though, is how sensitive this is. Because if you only end up seeing it after, arguably, the virological horse has bolted, and there's already a rip roaring outbreak in a particular town, it's not going to really add anything; whereas if this could be an early warning sign - the canary in the coal mine almost - and you get early warning of a variant coming or an outbreak about to erupt, then that would be really powerful.
Javier - Yes, you're right. Sensitivity is the key. You could increase it by looking at a higher volume of your sample, by increasing the frequency, and also by maybe doing a more targeted community surveillance in areas that, for example, you have detected new concerning variants. So you can go to smaller surveillance areas to increase the sensitivity of your sampling.
Chris - You obviously straddle the time when we've seen the emergence of things like the Kent variant. So have you been able to see that appear as a blip on your radar, and then begin to dominate - as it has done - during the course of doing these experiments?
Javier - Yeah, we have seen the variant very clearly appearing in our wastewater samples since early November. So at that time, this was not obvious from clinical samples yet. And then, the proportion of this variant increased really nicely - exponentially, actually, since then - to become almost a hundred percent by the end of January.
Chris - So it does look like there is the prospect to use this, and it could provide advanced warning of a threat that's coming.
Javier - Yes, indeed. And in fact, the way we've designed the processes would also detect those variants that have been firstly detected in Brazil and South Africa, which are quite worrying, including some mutations that we know seem to affect vaccine efficacy.
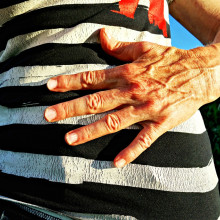
19:43 - Our super sensitive fingerprints
Our super sensitive fingerprints
Ewa Jarocka, Umea University
New research has emerged that suggests fingerprints may be good for more than catching criminals, and may in fact be responsible for the incredible sensitivity that humans have in their fingertips. Eva Higginbotham spoke to Ewa Jarocka, about her work published in the Journal of Neuroscience...
Eva - As burglar Harry Jackson found to his dismay, when he was the first person convicted of a crime in the UK based on fingerprint analysis, your fingerprints are unique to you. Your combination of whorls, arches, and loops arose while you were developing as a foetus in the womb, and your fingerprint - or pattern of papillary ridges - are yours for life. No-one's quite sure why exactly we have fingerprints; though some scientists have suggested that they provide either protection for our fingers, or increased skin sensitivity. But the evidence is still murky. Now, scientists that Umeå University have made a new, remarkable connection involving your fingerprints in your sense of touch.
Ewa - The fact that we can feel objects that we touch, or that we can feel even small vibrations - for example, if the table is vibrating and you put your fingers on it, you can feel it - that is enabled by our receptors that are located in the skin.
Eva - That's Ewa Jarocka, and she's part of a team who recently did an unusual experiment to try and get a deeper understanding of how the nerve cells in our fingertips work. The reason we can feel things at all is down to specialised proteins called receptors on our skin that respond to different kinds of stimuli like heat or pressure, which are then connected to our nerve cells which innervate our fingertips. Ewa was interested in how close together two points on your fingertip can be while still creating individual responses in your nerve cells; in other words, the spatial acuity of your fingertips.
Ewa - We knew that the neurons innervating our fingertips, they branch before they reach their end receptors. So you could imagine a nerve fibre as a trunk of a tree, and then you see multiple branches coming out of it, and each of them reaches a number of end receptors. We wanted to see how precise our touch can be, considering that we have those patchy fields of sensitivity, and if that was related to our fingerprint layout.
Eva - Essentially, you have these areas of higher sensitivity on your fingertips called receptive fields. And these receptive fields each activate a single nerve cell. Ewa and the team used a technique called microneurography to record single electrical impulses going through individual nerve cells.
Ewa - We inserted an electrode in the participant's arm, to a nerve that contains nerve fibres that innervate our fingertips. And when we were stimulating the skin, the impulses were triggered, and we were recording them. And we stimulated the skin with a pattern of raised dots that was wrapped around a drum, and we were spinning this drum with different velocities, and also with two different directions - towards the body and away from the body. During each drum revolution, we were able to scan one receptive field. And as a result, we created a map of sensitivity, so we could map where those branches were located in the skin.
Eva - What they found was that the spatial sensitivity of the nerve cells was the same as the distance between each of your fingertip ridges - 0.4 millimetres. This level of fine detail is why your fingertips are so sensitive in comparison to, say, the side of your arm. Importantly, they didn't test perception by asking the participants if they could feel a difference between two dots. But now we know that that level of incredible precision and detail is noticed and recorded by our nerve cells.
Ewa - Those neural responses were in the fingerprint ridges, they were anchored to the fingerprint ridges.
Eva - So next time you're enjoying the softness of a knitted blanket, or rummaging in the cutlery drawer for that specific spoon that you prefer, spare a thought for your fingerprints. They may be directly helping you feel your way through the world!
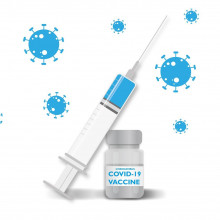
24:25 - Mailbox: Vaccines and boosters
Mailbox: Vaccines and boosters
Chris Smith, University of Cambridge
Stephanie has gotten in touch with a COVID question: Please could someone explain the mechanism of immune response over time to vaccines? Given that the booster needs to be at least a few weeks after the first dose... what happens in those intermediary weeks? Chris Smith has the answer...
Chris - What we don't know is what the perfect time to administer these two doses is. Because the only reason we used three weeks or four weeks initially was because that was the time set by the manufacturers in their trials, because they wanted to get the data quickly. And in fact, we've resorted to 12 weeks between doses because there's some data suggesting that actually the longer you leave it, the better the immune response is. And you might think, "well, why is that?" And the reason is that the immune response is not just a static thing. You don't just see something you want to respond to, make the response, and then call it a day. The immune system is continuously evolving, optimising, and maturing its response. So as more time goes by, the response that you make initially is slowly petered out and dissipates, and out of that emerges a much more focused, much better response, that's more finely tuned, but it takes time to get there. So waiting a bit longer between doses can in fact mean, when you do come back with the booster, you in fact then boost the really finely tuned, even better response you've now made - rather than a more broad, less finely tuned one you would have made if you stimulated with a booster too soon. And actually if you come back too quickly, with another booster too quickly after the first dose, you can in fact do the opposite and de-tune your immune response completely. It's finding that sweet spot that researchers are still trying to find at the moment.
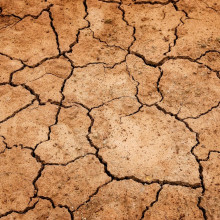
26:48 - The science of shaking
The science of shaking
Lisa McNeill, University of Southampton
When the ground starts to tremble, what’s going on below? Adam Murphy’s been finding out from the University of Southampton's Lisa McNeill…
Adam - Earthquakes can be absolutely terrifying events, showcasing that we are very small parts of a very big planet. The ground under our feet may seem solid, but it is split into loads of plates that gradually move around like the American plate and the Eurasian plate. But the planet isn't getting any bigger, so there's not a lot of room, and that means plates can crash into each other or scrape past one another along fault lines, but they don't move quickly. And a lot of stress can build up.
Lisa - The stresses build up on that fault plane and they build up over hundreds of thousands of years. And then when they build up a certain amount of stress that will get released rapidly over seconds and minutes. And that's what is the earthquake. It's that movement on the fault.
Adam - That's Lisa McNeill, professor of tectonics from the University of Southampton. Some earthquakes are bigger than others, and in a lot of places you would measure it with the Richter scale - where an increase of one on the Richter scale means an earthquake that is 10 times stronger. The 2011 Tohoku earthquake was a nine on the Richter scale, but it's not the only scale that's used.
Lisa - But in science, we actually use a different scale called the moment magnitude scale. And that's more scientific, it's better at measuring particularly the earthquakes at the larger end of the magnitude scale as well. And what that actually measures is the area of the fault that moves. And then the amount of slip that occurs during that fault movement as well.
Adam - And while the magnitude is important, it's not the only thing to consider.
Lisa - If you've got an earthquake that's being generated or fault under the sea, you potentially might generate a tsunami. The depth of the earthquake makes a difference. Usually the shallower the earthquake, the more impact it has on the surface and the human population. And also there are different types of faults that move in different ways. They tend to sort of generate some different scenarios as well. So there's lots of different types of earthquake. The primary factors are going to be how big a piece of fault moved and how much did it move? So that's what constitutes the magnitude. And the larger the earthquake, generally the larger the impact it's going to have.
Adam - When we talk about that 2011 Tohoku earthquake, you can't forget about the tsunami that followed. A giant wave crashing into the coast. It's what caused the problems with the Fukushima reactors. So where did they come from?
Lisa - A tsunami is where you're basically moving the sea floor in a certain way so that the water column above it moves. And that's what generates the tidal wave, the tsunami. So for a fault moving in an earthquake, it has to be above a certain magnitude to have a likelihood of generating a tsunami. And usually people sort of quote around a magnitude seven or seven and a half, something like that. And you do have to sort of have some kind of vertical motion of the sea floor. And that ground, that sea floor will have built up strain before the earthquake. And then it all gets released. And the whole sea floor moves, which basically moves the entire water column above that area. So in some of these very large earthquakes that tend to happen on subduction zones, which is where one tectonic plate goes underneath another, so these are where the largest earthquakes occur. So Tohoku-oki in Japan in 2011, is an example of that. In some of these cases, the area of the fault that's moving is hundreds by thousands of kilometres, or maybe sort of a hundred by hundreds by a thousand kilometres, something of that sort. So it's a really big area. So not surprisingly, you know, that does have an impact in terms of movement of the water column.
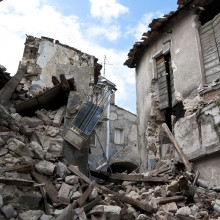
31:16 - Earthquake damage is more than just shaking
Earthquake damage is more than just shaking
Wendy Bohon, Incorporated Research Institutions for Seismology
Above ground, there are a lot more factors than just the geophysics, that determine the degree of damage that ensues in an earthquake. Wendy Bohon is in Washington DC where she’s part of the Incorporated Research Institutions for Seismology, and she spoke to Chris Smith about the different causes of earthquake damages...
Wendy - Not all buildings are created equal. So it depends on the type of material that the buildings are made out of and where the buildings are built. So there are certain types of soil that can be really bad during earthquakes. When seismic waves travel through rock, they go pretty quickly. But in areas that have soft sand and soil, like in valleys or on the edges of rivers, those areas actually trap the seismic waves. And so if you have a building that's built there, you'll shake harder. And for a longer period of time, which obviously is bad.
Additionally, certain types of building construction are more likely to fail during earthquakes. So the kinds of structures that have like car ports underneath, or parking lots underneath. Those can't take the side to side motion of the earthquakes. And often that soft first story will pancake causing complete destruction of the building. Additionally, wood frame structures are nice and flexible just like trees. So they're really good in earthquake shaking, but structures that may be more stiff that are made out of concrete or other types of materials like brick. Those can sometimes fail during earthquake shaking.
Chris - A lot to unpack there. Very interesting what you were saying about the location of where you put your building because what's underfoot really matters. Is it not just a question of - when you shake the ground, it's not just like the ground staying hard, can not the characteristics of the ground also change if you shake it up? I'm thinking we were talking about avalanches on the programme a year or so ago. And showing that actually snow, when you shake it, behaves quite differently than when it's just a snow pack.
Wendy - That's absolutely right. One of the things that we're concerned about in areas that have that soft sand and soil, but that also have a high water table, they can experience something called liquefaction. And this is where the ground turns almost into quicksand. So as the seismic waves pass through the ground, they push that water up towards the surface. This forces all those little pieces of sand and dirt apart, which means that the surface of the ground loses its strength. So anything that's built there or anything that may be standing there, like say a car, that will sort of sink down into the ground. Now it's not going to sit down and get totally swallowed, but you can imagine if your house is built on an area that experiences liquefaction and your whole house tips the side, even if your home doesn't fall down, that could still be a total loss.
Chris - Do we know roughly what fraction of the housing stock the world has are in those rather inopportune places? Because this is presumably something we've learned since. And we tended to live where it was nice to live rather than having done a survey of what was underfoot before we built a city there.
Wendy - Right! If only we had known what we know now! But we can improve those things moving forward. I'm not sure about the total amount of construction in the world that are built in seismic hazard zones. And there's lots of different areas that will experience landslides or liquefaction or ground shaking. But we can definitely make sure that we know what those hazards are. We can let the people that live in those areas know what they can do to help mitigate or solve those hazards. And we can build any new structures up to seismic standards. So we can take those older buildings also and do things called seismic retrofitting - things like strapping homes down to their foundation. So they don't slide off during earthquake shaking. Making sure that we put in shear walls, because when the earthquake passes through it doesn't just shake a building back and forth, it gives it an upward push, a sideways thrust, a downward drop, and then a sideways thrust in the other direction. So we need to have the building strong in all different directions. And we can do that even after the buildings have been built. So a combination of policy changes, making sure that we're putting money into the system so that we don't have to put money in after the earthquake happens to rebuild. And then also educating the people that live in those areas about what those hazards might be during the earthquake.
Chris - And are earthquakes made equal? As in, I know there can be stronger and weaker, but do they tend to all shake the ground the same way, or do they come in different varieties, which actually might shake the ground differently? And therefore it's all very well to say, well, we'll build our buildings to resist an earthquake like this, but because there are so many varieties, it could be really tricky therefore to defend against all those threats.
Wendy - The first thing is that we spend a lot of time talking about the big ones, the ones that affect, you know, really large areas, like say the San Andreas fault, all of California, something like that. But we're actually more likely to feel shaking or to have damage from smaller earthquakes because they happen so much more frequently. We need to think about the big ones and plan for those really, really big events. But we also really kind of need to think a lot about what's happening locally and plan for that. Luckily scientists can go and figure out exactly where the faults are moving, where that stress is building up. We can go and dig down into the fault zones to figure out when earthquakes happened before and how big those earthquakes were. So that we get an idea of what might happen and how hard the ground might shake in these places. That way we can go and work with engineers and architects and say, this is the type of shaking that's been felt here in the past. And this is we think we can experience in the future. So taking what's happened in the past and applying it to the here and now I think will make a big difference.
Chris - That's certainly true in countries that have the resources, the wherewithal, and they don't have the pressures on the environment, the climate, food supplies, and so on to do that where you can make a rational choice. But in many places, as population climbs we're finding that people are resorting to living in areas that were a second choice previously, and that often does push them into the path of danger, doesn't it?
Wendy - It really does. What we know for certain is that disasters do discriminate and the people that are the least able to prepare for those disasters are often the ones who are going to be the most impacted when they happen. And so we really need to take into account vulnerable populations and the equity of how we're doing our communication about earthquakes, our communication about what those hazards are and working within populations that may be more vulnerable. Working with countries that may not have the infrastructure or the resources to go in and do the types of improvements that will really help to save lives and property during these big events.
Chris - Well, what then must scientists bear in mind? Or what must be the bottom line in terms of what we need to communicate to people going forward about earthquake threats and how they're likely to impact on us in the years ahead?
Wendy - Earthquakes can happen anywhere. We need to be ready when they do happen. And know that scientists are working very hard on your behalf. This is our science and we love it. But we recognise that these kinds of events impact people's homes, their businesses, it affects their lives. And that matters to us. We are working as hard as we can to make the changes that we need to make in order to keep everyone safe.
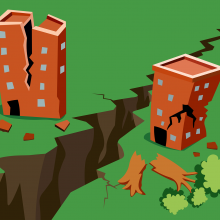
38:46 - Protecting old buildings from earthquakes
Protecting old buildings from earthquakes
Pierfrancesco Cacciola, University of Brighton
It’s not earthquakes that kill people, buildings do. And architects are now designing buildings that are extremely earthquake resistant. The Fukushima nuclear plants, after all, withstood the 2011 Tohoku earthquake in Japan just fine, it was the ensuing tsunami that caused the problems. But constructing new buildings is only part of the solution: we also need to protect existing buildings, including those that are of historical value. Luckily there is a way to do this, as Adam Murphy has been hearing from the University of Brighton's Pierfrancesco Cacciola…
Adam - An earthquake can level a city so a lot of effort is put into trying to protect buildings. There's plenty of options that can be done to new buildings - you can put in large weights which shake in such a way that it damps down the buildings own shaking, or the whole structure can be reinforced. But what about buildings that are already there that can't be retrofitted? Maybe there isn't enough money or it's old enough to make that tricky. You don't want to be drilling into the pyramids after all. Well, Pierfrancesco Cacciola from the University of Brighton has a potential solution - the vibrating barrier or VIBA
Pierfrancesco - The vibrating barrier is essentially a big box in the soil with a mass that is able to oscillate inside the box. The device is designed to protect nearby structures without touching them. So practically you need to see this device as something completely detached from a structure but next to it, and because of this oscillation of the mass it’s able to absorb the energy from an earthquake, and to reduce therefore the action of the ground motion underneath the structure and reduce the vibration of the structure that you want to protect.
Adam - It is potentially quite a simple solution to a very difficult problem, but how does it work?
Pierfrancesco - It has been proved a very long time ago now that when an earthquake arrives a building reacts in a different way if it is on it's own or built next to another one, because practically the buildings hold each other to the soil. And this phenomenon is called 'the structure-soil-structure' interaction. A structure that is built next to another structure will have its dynamic response altered, and altered means either larger or smaller. There is a dummy structure, designed in such a way, the structure that you want to be protected will have a reduced vibration, a reduced dynamic response. You want to create a weak link in a chain if you want, so to create an element that is able to vibrate more, and will be, if you want, weaker, will absorb more energy and because this energy is absorbed there it will be a reduced from the energy underneath the structure that you want to protect.
Adam - So you can think of it as a big, heavy cube, say, that can shake, maybe it's on springs or it can oscillate in some way. And it's connected to the structure you want to protect. When the earthquake's waves come rolling in this cube shakes, and it absorbs all the shaking, all the energy that would have gone to destroying the building, keeping your buildings standing and safe. Now the cube does need to be very heavy, about 50% of the mass of what you want to protect, but you could use a lot of smaller ones. And Pierfrancesco has been working on getting the mass required down to something really feasible. So given that the vibrating barrier has been in the works for a while now, and these things do take time to develop, that's understandable, what state is it currently in?
Pierfrancesco - So we are working on two other projects. One is a project about the protection of the cultural heritage in Egypt. And we have, as a case study, the Zoser pyramid, one of the most ancient pyramids in Egypt in Sakara, and the Citadel of Saladin in Cairo. We are working at the moment on the seismic protection to the vibrating barrier of the Zoser pyramid. Our preliminary results are quite promising. And another project that is a PhD scholarship on the seismic protection of historical buildings with the vibrating barrier - in this advisory group there are colleagues from the ministry of the cultural heritage in Greece. And another case study is the seismic protection of the Messina Cathedral in Sicily. The Messina Cathedral has the bell tower that is the largest astronomical bell tower in the world. So we started to protect this bell tower that has been already damaged by an earthquake in 1908.
Adam - And Pierfrancesco is quietly confident about the future of the vibrating barrier.
Pierfrancesco - I believe that this technology might provide a different angle to protect existing buildings from our earthquakes. Honestly, I do believe that when the first vibrating barrier will be built, quite few more will follow.
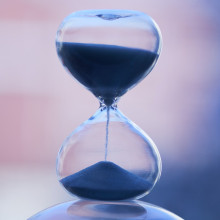
Predicting future earthquakes
Joanna Faure-Walker, University College London
Protection against the effects of earthquakes matters of course, but if we could predict their impending arrival, that would be even better: we could evacuate people; put rescue services on standby; prepare to turn off gas or electricity supplies if necessary, and so on. But, regrettably, it’s not that easy. And to explain why, Chris Smith was joined by UCL's Joanna Faure-Walker…
Joanna - The problem is it's, as you mentioned, very difficult to actually predict an earthquake. So when you're talking about prediction, you're saying, you know, how big will the earthquake be and exactly where and when is it going to occur? So is it going to be on Tuesday at 5:20 PM and magnitude 8 on this particular fault? We're nowhere near that. The problem is to be able to do that - let's just consider one fault in isolation at the moment. If we wanted to be able to predict when the earthquake would occur, we would need to know how large we expect the earthquake to be, so how much movement on that particular fault do expect that to be? And how long would it take to build up the stress required for that amount of movement? So how fast is the fault moving? We'd also need to know how much of that movement is taken up, what we say seismically, so in earthquakes, versus aseismically, so not in earthquakes. And then we would also need to know when was the last earthquake on that fault so we know where we're starting our timer. Now, all of those things are actually very difficult to measure. We know some of them on some faults, we actually know almost all of them on particular faults, but in most places in the world, most of those elude us. Even if we did know all of those things, it's more complicated than that because faults don't behave on their own. They're not isolated structures, they interact with each other. So the forces that are governing the timing of these earthquakes is just more complicated than at the moment our models can deal with.
Chris - I was going to ask you - do faults talk to each other? in the sense that if you've got one that starts to unzip or move, does that actually have an impact or knock on effect at encouraging another fault to unload itself as well? Does it transfer the stress elsewhere, if you like?
Joanna - It does exactly that. I like your expression there of talking to each other. When an earthquake occurs on a fault, we lose the stress on that fault. It releases the stress there. But it also is causing movement throughout the crust, that's where we're standing on now and that's where these earthquakes occur. So other faults will either be accelerated or you can actually bring delays so you can slow down that rupture. So the problem with this is the idea you'll sometimes hear about recurrence intervals on faults - so the average time interval between earthquakes. But if we've got all these interactions taking place, it's not a regular time interval and so it's much more difficult to predict again.
Chris - It becomes a massive, massive problem doesn't it with so many different dimensions to it. Have new tools helped us though? Because we've got things like GPS that can make very accurate movement assessments of how the land is moving that would previously have been very difficult to do at the sort of scale that we can now do them. Does bringing more data to the table help with these sorts of predictions?
Joanna - It really does. It really helps us understand the fundamental mechanics - so how fast are these faults moving? But the problem is we're looking at timescales here. Now as a geologist, when I talk about short term time periods, I'm talking about hundreds or even maybe thousands of years. So as a geologist, that's a short time period. Now these GPS records that you just referred to can look at years or tens of years, but they're not going back hundreds of thousands of years. Now, if we consider an individual fault, they can move at parts of millimetres or centimetres per year. So about as fast as our fingernails grow. How long would you need to not cut your fingernail before you could have, you know, a metre or even 20 metres of slip in one event? So on an individual fault, it may be hundreds or thousands of years between individual earthquakes. And with these modern methods of analysing the movement, yes, they're giving us a lot of information, but we're looking at such a short time period compared to the length of what we call the seismic cycle.
Chris - Are there any other kinds of markers that might be proxy markers of energy that's being stored in compression in these faults that's slowly building up? Because, you know, I know people say the animals change their behaviour ahead of earthquakes. Now this might just be attaching significance to coincidence might'n it because about 10 years ago there was this lovely paper - unfortunately it came out around the time of April fool's day which meant everyone kind of said they thought it was a joke, but it was a bunch of toads that just disappeared from when they would normally be mating around a site in Italy that then a few days later had a huge earthquake. And the researchers there speculated perhaps there were changes in the Earth's ionosphere, the pattern of how the earth guides radiation round its outer part of space. Are there any markers we might be able to plug into that would point us towards a critical stress building up at certain fault points?
Joanna - There are some ideas and some of them are more promising than others. I mean, the problem with animal behaviour is we are yet to see a statistical study. Something like the toad behaviour, or dogs barking, things like this, if you actually monitored the toads every single day of the year, and you kept doing this year after year, and you actually said that there was statistically significant different behaviour on those dates, then you might be able to start using some of these methods. But as yet, I do not know of any studies where they actually look at these. So you hear people saying things like the toads moved or the dogs barked, and it's like, well, yes, toads move and dogs bark. So I don't think those are particularly promising at the moment. But what we can do, and a lot of the work that I'm involved in, is trying to look at long term rates of deformation. So geological timescales for thousands of years, tens of thousands of years, and we can use markers on the longterm where we look at how rocks have been displaced relative to each other, to try and understand how fast are these faults moving in the long term? And then compare these to things like the GPS records to see where are we in that cycle of stress building up, and is there any helpful information about imminent failure.
Chris - And are we better at doing this these days? Or are we just better at knowing we're no good at it, if you see what I mean.
Joanna - We are better. So we are producing what we call probabilistic seismic hazard approaches. So this is where we use probabilities - so how likely is an event to occur in the next 5, 10, or even a thousand years, depending on what you're interested in, and on those we are making very good progress. It helps us make relative risk decisions. But in terms of actually saying whether we expect an earthquake at 5:00 PM on Tuesday we still have a way to go.
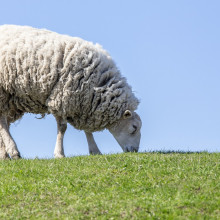
52:38 - QotW: Do animals get bored eating the same thing?
QotW: Do animals get bored eating the same thing?
Katie Haylor's been looking into animal boredom with the help of the University of Waterloo's James Danckert...
Katie - Well Douglas, to my embaaarassment - sorry - I’m not really sure.
Luckily, members of our forum - nakedscientists.com/forum, are far less sheepish at venturing an answer.
User Alan Calverd reckons it depends on the animal. Alan says that pigs, dogs and chickens are very experimental within the human-dominated environment. Other apes tend to graze a variety of vegetation and the occasional small animal.
Some animals famously only eat a very limited range of food - like koalas for instance. Do they get bored on an exclusively eucalyptus diet? I would love to know - if anyone’s done that study, please get in touch!
Back on the forum, user Evan AU hides food around the house each evening for their pet pooch to find - and they reckon the dog has a great time!
So I put Douglas’ question to boredom neuroscientist James Danckert from the University of Waterloo in Canada.
James - Whether sheep get bored with the same old patch of grass or not is hard to say, but animals do get bored. If you house animals in bland surroundings – pens or cages with little to nothing for them to do – their behaviour when they get out of those environs is what you would expect from a bored animal.
Researchers in Canada did this with mink and showed that the mink housed in bland cages were quick to approach all kinds of objects once they were released. The researchers reasoned that if the mink were depressed or apathetic they would have shown little interest in any new objects. But if they were bored they would quickly approach anything, even things they would normally avoid – like the smell of a predator.
And that’s precisely what they found. They even showed that the bored mink overate treats at the end of their experiment. And we see this in humans too – overeating out of boredom!
Whether all animals feel boredom is an open question. If we assume that boredom’s function is to signal the need to explore your world for something new and valuable to you, then I suspect it won’t just be mink who feel boredom.
Katie - I wonder if boredom explains why one of my cats just loves attacking my slippers whenever she comes across them in the hallway? Thanks very much James for enlightening us on that one.
Next time, we’re computing this chromosomal conundrum from Mattie.
Mattie - If humans have too many or too few chromosomes, it can cause them to be infertile. So how do different creatures get different numbers of them? For example humans normally have 46 chromosomes, but mice have 40.
Comments
Add a comment