The Science Too Hot To Handle
The Olympics is finally upon us and from going for gold in the tropical Rio climate to boosting the efficiency of jet engines, our ability to cope in high temperatures could make the difference between falling or flying. This week on The Naked Scientists we're exploring the many ways in which humans, and machines, can handle the heat. Plus, which country tops the charts when it comes to height? Also, we'll hear how tomatoes hold the key to fending off a deadly parasite.
In this episode
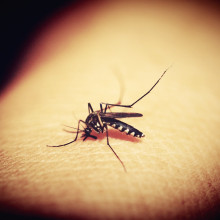
00:54 - Millions at risk of Zika infection
Millions at risk of Zika infection
with Professor Andrew Tatem, The University of Southampton
As the US declares its first "home-grown" cases of Zika in Florida, we've learned that up to 90 million people, including over one and a half million childbearing women, may be infected across the Americas as the initial wave of the Zika epidemic unfolds. Andy Tatem from the University of Southampton took Chris Smith through the numbers...
Andy - Back in February, WHO declared Zika as a public health emergency, but there were no real estimates at the time of the scale of the problem and that's useful for decision makers for planning surveillance, for working out outbreak response, for working out what the demands might be on their health services. Normally, to produce these kinds of estimates you would make use of data that's coming as the endemic is going on, but often using those traditional approaches and using that data the damage can be done because the epidemic is ongoing. So we leveraged data relationships from previous epidemics that have been spread by the same mosquito, viruses such as dengue and chikungunya. We know they have relationships with things like temperature that we can utilise to be able to make predictions across the region that we're interested in, which is the Americas in this case.
Chris - And because these viruses are inhabiting the same territory, they're spread by the same mosquito vector, the same factors that influence the spread of those viral relatives will probably impact on the spread of Zika. And that means you can then make more realistic predictions about where it might go and over what sort of timeframe?
Andy - Yes, certainly. We're assuming that Zika will be spread in a similar fashion, we know it's spread by the mosquito that has spread previous similar viruses, so we can make those assumptions at the start of the epidemic. And, obviously, as the epidemic goes on we'll have more data on Zika itself and see any differences that exist. But in the rapid spread of this virus, we need answers quite quickly to ascertain the size of the epidemic and be able to react.
Chris - The numbers which were being put forward at the time when the WHO made its announcement and, subsequently, have been really rather large; we've been talking about millions of potential cases or exposed individuals. What does your approach suggest - are those numbers reasonable?
Andy - Our estimates are of the scale of 93 million people across the first wave of the epidemic over two to three years and of those 93 million infections, we're estimating around 1.6 million childbearing women.
Chris - And where are these 93 million people?
Andy - They are spread across the Americas, and we were making predictions at 5 by 5 kilometer grid cells. So we are making really upper limit predictions of, if the virus made it to that location, what was the kind of upper limit that you would expect in an epidemic.
Chris - Where are the hotspots?
Andy - The hotspots are really around Brazil; it has suitable climate; we know it has high densities of the Aedes aegypti mosquito that spreads Zika. It's also in some of the Caribbean islands and there's been good evidence of big outbreaks of these kind of viruses on small islands before.
Chris - And if we extrapolate the number of infections that you're seeing, what are the implications for what people seem to be, rightly so, most worried about with this virus which is its impact on women who are pregnant?
Andy - I think there's still a lot of uncertainty there in terms of the relationship between getting an infection and birth defects. We are estimating here around one and a half million women giving birth who may be infected. There's a range of estimates of the proportion of those that might give birth defects and those numbers have been quite widely varying and are very small samples at present. So I think, as the epidemic goes on we'll know a lot more about that.
Chris - And what's your thoughts on the situation in Brazil given that we're about to start the world's most important sporting event there, and something like half a million to a million people will come to Brazil to support and compete?
Andy - I think there is a need to be vigilant, to improve and keep up surveillance. There's obviously a need for mosquito control in some of the most visited and highest mosquito density areas, and I think there's still a lot of uncertainty. Obviously there is a worry about people coming from all over the world taking not only Zika back, but other diseases and spreading those across the Americas. And at least now we have some estimates of, if that virus is taken to new locations of what might be the scale of the local epidemics that might occur.
Chris - Because, of course, the data you're using in order to make your predictions is based on things like dengue virus, but it based on the natural cycle of dengue, not when there's an Olympics going on, isn't there? So might there be a bit of a change to the outcome because of the Olympics?
Andy - Yes. I mean we don't account for human movement here. We are estimating if the virus were to get into a location, what might be the scale of that epidemic. If we have good information on the number of people who are moving between locations; if we have good data on how that's connected to other parts of the world in terms of numbers of people coming from different countries, we can then extend to make estimates of how that virus might spread to new locations, and not only Zika again but other diseases.

06:37 - Testing for sports doping
Testing for sports doping
with Kerry Parker, Select Science
Sticking with our Olympic theme, the headlines have been hogged by Russia narrowly avoiding a blanket ban after several athletes were found to have taken performance-enhancing drugs. But how do organisations like WADA - the world anti-doping agency, test for these substances? Georgia Mills spoke with Kerry Parker, who's the editor in chief of the website Select Science; she's recently been to see the testing labs in Rio...
Kerry - You're going to several rooms; firstly where they bring in the samples. So what happens is urine or blood samples are collected from athletes in the field, they're couriered in, they're given unique codes so nobody in the laboratory can actually know which athlete has which sample, and then those samples are processed.
There's an A and B sample. So the A sample is processed and the B sample's always kept closed, so that's a secondary sample that's only opened in the future if there needs to be some retesting. So what happens next is the samples are processed and they go through different types of analytical procedures and, essentially, what they're doing is they're looking for banned substances or substances that look like those banned substance. They're also looking at unusual metabolomics or unusual results in the test as well.
The Rio laboratory I've just visited recently was absolutely amazing. They had a huge room full of instruments and it's a very unusual set up compared to any other kind of laboratory you'll find anywhere in the world. They're going to be processing over 6,000 samples in about three weeks over the Olympics; that's about 300 to 400 per day and they have to get those results out within 24 hours. Years of preparation go into setting up the equipment, training the staff to run a 24 hour lab.
Georgia - In terms of actually testing for these substances, what do they do - do they sort of run it in a machine or look at it under the microscope?
Kerry - Generally, they're doing analytical tests; they're looking for particular compounds in say a blood or urine sample. You might use a science called 'chromatography' and 'mass spectrometry.' This will help firstly separate molecules in a sample like urine and then once those molecules are separated, that science will be able to identify what those molecules are by their weight. It's a very, very accurate type of science, and from that report the scientist can see if particular molecules are present or not in that sample at a very, very low level of detection.
And so what's really interesting about this whole world or doping is that those athletes that do decide to dope, they may be utilising new designer drugs or substances every year. So part of the work of this laboratory is not just to look at what's been banned but also look at are there any other new drugs that are being utilised at this time.
Georgia - There seems to be a lot in the news about doping, especially recently I guess with the Olympics coming up, but is it going up?
Kerry - I don't know that we can say that. All we can say is that the level of detection is increasing, the scientists can detect at a much better level, and they can also now look forward and look back as well. So I think the science is absolutely there, and the detection is there. Perhaps that's why we're hearing it more in the news and, obviously, around the olympics these stories are always really important, and we mustn't forget that athletes are tested all the time actually. It's not just around these games, they are often tested in between competitions as well. Many of them have what's known as the 'athlete biological passports'. They're collecting data and recording and recording data over time as well. So I think, when the Olympics comes round it's a topic that everyone's interested in, but it's something that's happening in the background all the time in athletics and sport.
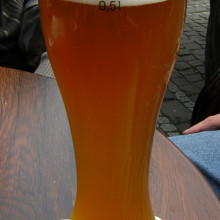
10:36 - Myth: Asians can't break down alcohol
Myth: Asians can't break down alcohol
with Dr Kat Arney, The Naked Scientists
Kat Arney is back with her mythconception and this week she's been busy researching in the pub...
Kat - If you're from an Asian background, or if you've ever been out boozing with Asians, you've probably heard of 'Asian glow', and maybe even seen it in action. It's the flushed red face that some people get when they drink alcohol, along with other effects such as a fast heartbeat and a raised temperature after just one or two drinks. This isn't just restricted to Asians, but it is much more common in people from places such as Japan, China and Korea, affecting up to a third of the population there. As a result, many people think that 'Asian glow' is due to a genetic inability to break down alcohol. But in fact, this is a myth - or, at least, the truth is a bit more complicated.
Let's, err, break it down further. When you drink alcohol, whether it's in beer, wine or spirits, it gets broken down in the body, with the products ultimately ending up being used for energy - or stored as fat (it's not called a beer belly for nothing...). The enzyme that starts the breakdown process is called alcohol dehydrogenase, or ADH. What it does is removes an atom of hydrogen from the chemical structure of ethanol - that's the scientific name for the alcohol we drink. This leaves a related chemical called acetaldehyde - and it's here where things get nasty. Ethanol itself isn't really that bad for your body - apart from the health risks of excessive drunkenness, including accidents and other risky behaviour. But acetaldehyde is relatively toxic, and can have several negative effects on the body, including the unpleasant symptoms of a horrendous hangover the day after, as well as dilating blood vessels in the skin and leading to the famous Asian glow while drinking.
Because of this toxicity, there's another enzyme called aldehyde dehydrogenase, ALDH. Somewhat confusingly, this actually adds an oxygen atom back onto the acetaldehyde, creating acetic acid - the main component of vinegar, and a chemical that our bodies can easily use for making energy. If you imagine the body to be a bit like a funnel, alcohol comes in the top, alcohol dehydrogenase breaks it down into acetaldehyde - which is toxic - and aldehyde dehydrogenase gets rid of that by turning it into acetic acid. If both these enzymes are working properly - and you don't overload the system with too much booze - then you can easily deal with a couple of drinks. But rather than having a problem with the first step of the process - breaking down ethanol - people who experience the Asian glow, or alcohol flush reaction as it's more formally known - have a genetic variation that means their aldehyde dehydrogenase is either ineffective or less efficient. So acetaldehyde quickly builds up, causing the rosy cheeks.
There's another part to the story too. Many Asian people actually have more efficient versions of the first enzyme in the chain - alcohol dehydrogenase - which means that they make acetaldehyde relatively quickly as soon as the first glass of booze hits them. And as well as being embarrassing for some people who suffer from it, this can have serious health effects. The genetic change has been associated with an increased risk of certain types of cancer - particularly cancer of the oesophagus, or foodpipe - due to higher levels of acetaldehyde that can cause damage to cells and DNA. Unfortunately there aren't any really good ways to counteract this, and the best advice is just to cut down on booze.
And finally, there are some bodily effects of alcohol that you can't blame on your genes at all - and that's the bad drunken dancing. Anyone for the Macarena?
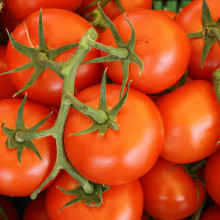
14:40 - Tomatoes to the rescue!
Tomatoes to the rescue!
with Dr Markus Albert, University of Tubingen
A parasitic plant that devastates crops in some parts of the world may now have met its match thanks to the household tomato. Cuscuta reflexa invades the stems of its hosts and steals water and nutrients. This damages the yield and also leaves the victim susceptible to infection by other plant pathogens. But, by chance, tomato plants have naturally evolved a mechanism to detect the parasite and, when it tries to invade, they kill it. Markus Albert, who made the discovery, has now uncovered how tomatoes do this, and thinks it might be possible to endow other crop plants with the same defence system, as he explained to Chris Smith...
Markus - This is a plant but it's also a parasite which attacks other plants. This plant has no roots, it has no leaves, so it requires nutrients from other plants. It grows inside the stem of another plant and then it starts to withdraw water, nutrients, and also carbohydrates.
Chris - What does the parasite look like then this parasitic plant, before it gets inside the host plant?
Markus - So the parasite is a winding plant that winds around stems of host plants. Before it starts to penetrate it has a special organ called 'haustorium' - a kind of a root that grows inside the host plant, and then it connects directly to the tissue where water flows. So it makes a continuous tube from the host to the parasite and the parasite is getting everything out.
Chris - What sort of impact worldwide does this class of parasite have on plants that we judge to be important - crop plants, for example?
Markus - In Southern Asia it's a very dangerous plant in the soya bean cultivation. Even for some tomatoes it can be very dangerous or it decreases the crop yield tremendously. And also in coffee cultivation in can be a really huge problem. The biggest problem - you cannot fend off this parasite by using herbicides because, otherwise, you would also kill the host plant or the crop plants.
Chris - How does it spread then - does it make flowers at some point and set seed?
Markus - It makes flowers and this flowering time is somehow synchronized with the host plant. These parasitic seeds, they can stay in the soil for years.
Chris - And are all plants, as far as we know, susceptible to this parasite or are there some that have evolved to fend it off?
Markus - Nearly all plants are susceptible to this cuscuta species, but we noticed that the cultivated tomato is resistant against this parasitic attack and there we observed an active defence. The site where this parasitic penetration organ aims to penetrate the tomato stem, the surrounding tissue becomes kind of brownish, and what we then observed when we made a cut through the stem, this haustorium could not grow inside. And very important, about two weeks later, the parasite dies.
Chris - So just by chance, it would appear, the tomatoes that we grow because we love to eat them appear to have some kind of mechanism that can fend off this parasite - it stops it plugging into the vascular system of the plant. How did you then pursue that to work out how it's doing that?
Markus - We also noticed that a wild tomato species was susceptible. Other people crossed the two tomato species and provided us a set of different tomato strains with a diverse genetic background, and these were all tested and this helped us to further characterise and identify important components.
Chris - I get it. So by comparing a strain of plants that are susceptible to the parasite with a strain of plants that are not susceptible to the parasite you can then ask well, what are the differences between the two? Because the differences must hold the key to that resistance and then you can begin to pick through and see what exactly is missing from the plant which is susceptible and that tells you. So what is it - how is the tomato plant fending off the parasite?
Markus - We mapped a certain region of this tomato genome and we ended up with just one important component and this is a kind of receptor mechanism. This receptor could detect a molecular signature from this plant parasite and then it works like a switch and starts a kind of signalling cascade that leads to this defence.
Chris - Would this tripwire system be amenable to being transferred to other plants that are currently susceptible to the parasite? So could you put the same machinery into soya beans, for example, so if the parasite tried to get in there, then the soya bean would also be able to fend it off?
Markus - So, I think this is something important we aim to do. What we initially tried - we transferred this machinery to two other plant species and there we could see the resistance increasing against this parasite. And now it will be an important aim to transfer this also to important crop plants, for example, soya bean or some others.

20:40 - Which country is tallest?
Which country is tallest?
with Dr James Bentham and Professor Majid Ezzati, Imperial College London
If you've been to the Netherlands lately, you might have noticed how tall many of the people there are - especially the men. That's because they're now the tallest nation in the world. But it wasn't always like this: the largest ever analysis of height measurements from nearly one and a half million people across the globe and spanning more than a century, reveals how height has changed. Kat Arney spoke to Imperial College's James Bentham and Majid Ezzati who were part of the team behind the study, to get the 'low down' on the world's height over the past hundred years...
James - So the tallest people were in Scandinavia, they were in the USA, Canada, Australia, and so on, and also some Pacific Islands. The shortest people were in parts of Central America, they were in the Middle East, in Southeast Asia. The average height of men was around 5'7" in the tallest countries; the average height of women was much shorter.
Kat - And now, how has that changed - again where are the tallies and where are the shorties and how much have they grown?
James - The tallest people now are in Europe, so the top ten countries for both men and women are European countries. On the other hand, the countries that have grown are spread round the world. So the countries that have grown the most are in East Asia, so Japan and South Korea have grown particularly dramatically. Iranian men are much taller and then there's been a lot of growth in southern Europe as well.
Kat - So this hasn't been across the board - the whole world is getting taller. There are unevennesses?
James - There are unevennesses, yes. So every country in the world has grown taller but some have only grown taller by a matter of a centimetre or so. Whereas other countries, for example, women in South Korea are 8" taller than they were.
Kat - Wow! I wish I could be 8" taller. And are there any countries that are actually getting smaller?
James - There are countries that are smaller than they were 30 years ago, or 40 years or so.
Kat - So they had a peak and now they're getting shorter again?
James - Yes, particularly in Sub-Saharan Africa - we see this in various countries there. They reached a peak and since then their height has declined by 5-10 centimetres.
Kat - So Majid - we've heard how the data has changed - how these trends have changed. How some countries have got taller and then plateaued, some countries are getting much taller and some have got taller and then dropped off. What's behind these patterns of changes?
Majid - We do know from decades of work in nutrition and physiology that height is really a matter of nutrition, environment and the care that they get. And in some sense, the changes, the improvements and the variations reflect better and worse social, nutritional, and environmental conditions during pregnancy, in early childhood and adolescence, and they reflect that accumulation of that advantage versus adversity.
Kat - As a geneticist I know there's a certain proportion of height that is in the genes and a certain proportion that's to do with the environment. How does that balance out in different countries - do we think that maybe some countries have better height genes than others?
Majid - Sure. If we take a snapshot at one moment in one population, genes are hugely important and, again, some of the best studies seem to show that 20/30% is due to genes. But genes don't change very fast over time, it's really environment and it's really nutrition. While there may be variations in genes between different countries, the belief is that it's role is much smaller than differences in environment and nutrition.
Kat - So if it does mostly boil down to nutrition, what kind of foods are important?
Majid - So we should remember that nutrition isn't just about food. It's about the conditions in which the foetus grows, so the mother having a good environment and good nutrition, and it's about maintaining nutrition. Food does matter and animal products, animal protein, and dairy are quite helpful for growing taller. They include the components and nutrients that help with growth but it should also be the case that those nutrients are maintained. So a child that lives in a home that doesn't have clean water and actually gets repeated episodes of diarrhoea, they lose any nutrition that they would be getting. Or a child that doesn't have ready and high quality access to healthcare so that they are treated when they get an infection, when they have an illness, those things matter.
Kat - Why is it important? Obviously it's very interesting from a data, nerdery point of view to look at height and see how things have changed and we can draw some cool graphs, but why does height matter - what does it reflect?
Majid - Greater height is associated with longer longevity and better education and economic performance. So it's really the connection between early life conditions and later life health. For that reason it says a lot about how society thinks about its future.
Kat - So James - just one final question. I am 5' tall (about 152cms) - where in history would I have been tall?
James - In 1914, you would have been tall in Guatemala because the average height of Guatemalan women then was 4' 7", and you'd still be taller than average there because the average height was 4' 11".
Kat - So basically, I need to move to Guatemala?
James - Yes! Essentially that's the answer.

26:36 - The mystery of Starlite
The mystery of Starlite
What sort of material could withstand high temperatures without burning or giving off toxic fumes? We take a look back in time to find out...
This torch here is producing a temperature of 1200 degrees Celsius. Now try cooking an ordinary egg like that and, in a very few seconds, the results would be quite an explosion. But I'm going to leave this torch here, blowing on this egg for a couple of minutes before we crack it open and it ought to survive the inferno because it's coated with a remarkable new plastic...
Connie - That was the BBC's Tomorrow's World programme, on TV, back in 1990. And that 'remarkable new plastic' being demonstrated live, was known as Starlite. This coating, allegedly, could withstand intense heat; and it didn't catch fire and it didn't release toxins, making it a very attractive industrial proposition. In fact, the egg coated with it survived a full five minutes under the blowtorch flame...
So, how's it doing? Well it hasn't broken up at all and you can see on the front here it's glowing red hot. But just watch this, if I turn the flame off (and remember that it was producing 1200 degrees Celsius), and I take that charred bit and I put it flat in the palm of my hand, it only just feels warm. And if I then crack it open, what's more the egg hasn't even begun to start cooking...
Chris - Perhaps just as remarkable as Starlite's properties was the story behind it - it was created in the 1980s by former hairdresser Maurice Ward. Ward's inspiration for such an invention followed an aircraft fire in which 55 people died through exposure to toxic fumes released inside the cabin. So, could he create a plastic which couldn't catch fire?
He set about concocting different formulations using the food mixer at home. He eventually stumbled on a few recipes which passed formal tests at the chemical company ICI. But it wasn't until his appearance on Tomorrow's World that things really started to heat up.
Connie - A series of tests by various government agencies was begun. They found Starlite to have little problem resisting nuclear blasts of ten thousand degrees Celsius and the force of 75 Hiroshimas. It was virtually unscathed when subjected to an intense laser beam. The scientific community was baffled. It wasn't clear how Starlite could do what no other material had done before. But what was clear, was its potential.
Chris - Coating something in a thin layer of Starlite could make it resistant to fire and radiation, while also providing remarkable thermal insulation. Rumoured bidders such as NASA and Boeing were eager to get their hands on a sample, but Ward had other ideas. He refused to let Starlite out of his sight, and he kept its recipe a closely guarded secret. All he would reveal was that it was made up of 21 organic polymers and co-polymers, and small quantities of ceramics. The true secret was apparently only held by Ward and some close family members.
No. We don't supply you the formulation. If we give the world the formulation - that's exit us!
All we're saying really is that I'm protecting my material... and you ain't gonna pinch it!
Chris - Now before he would give any company a licence to the material, Ward required them to sign an agreement promising not to reverse engineer it. Dozens of corporations never made it past the negotiations stage, and enthusiasm for Starlite petered out.
So what was the mystery behind the substance? Well some say Ward was being greedy and selfish, others say he didn't want it to fall into the hands of defense companies. Or was it just a big hoax? Unfortunately, we're never going to know the answer because Ward passed away in 2011, and he took the secret of Starlite with him - it's never come to light.
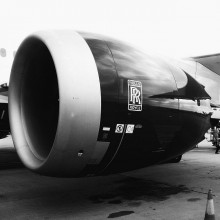
30:23 - How do you stop a jet engine melting?
How do you stop a jet engine melting?
with Neil D'Souza, Rolls-Royce, Cathie Rae, The University of Cambridge
The inside of a jet engine reaches temperatures hotter than molten lava! These temperatures are also above the melting point of the metal turbine blades which make up the engine interior. These blades are forced to spin by the expanding hot exhaust gases travelling through the engine. They extract energy from the gas stream and use it to drive the large fan that are visible at the front of the engine, and this is what produces most of the thrust. Clever materials science and engineering keeps these blades from melting. Claire Armstrong has been discovering how...
Claire - At any one time, there are a million people airborne all across the world. The Airbus A380 (a double-decker long haul aeroplane) can weigh up to 590,000 kilograms when full of passengers, luggage and fuel. So how is it possible to lift up something so heavy and keep it floating in the sky?
The answer lies in the aircraft's incredibly powerful engines. To find out more I went to Derby to visit Rolls-Royce, one of the world's leading manufacturers of aircraft engines, and who have been manufacturing these engines for over 100 years. I was joined there by Neil D'Souza who works with the blades that make up the engine. Firstly I was curious to find out how do these engines actually work?
Neil - Effectively what you do is suck in air through the fan, you compress the air - that increases the pressure - that increases the temperature. And then you combust it with fuel from the combustion chamber, and then the hot gasses that pass thereafter are at about 1600 degrees as they enter the turbine. They begin to expand and the energy that's extracted from the gas stream then is used to drive the compressors etc., and then you basically get a thrust. And the thrust is the most important part, which effectively keeps the aircraft flying.
Claire - You mentioned 1600 degrees Celsius. How on earth can you manufacture turbine blades to deal with such extreme temperatures?
Neil - The normal melting point of the nickel blade alloys that we use in the turbine is typically about 12-1400 degrees. But what you do, and this is the clever bit, is you actually cool these blades. You have internal cooling passages, which effectively has air that flows through and it's about 7-800 degrees. And this cooling air then exits from small little minute holes that have been drilled on the surface of the blade and this air then forms a kind of a film on the surface of the blade, and this technology is typically called a 'film cooling.'
What you also do - you coat these blades and typically use something called a thermal barrier coating. The thermal barrier coating, effectively, is ceramic, typically about quarter of a millimeter in thickness, but they have got very, very low thermal conductivity. So, effectively, even though the gas stream is at a much higher air temperature, the effective metal that exists beneath the thermal barrier coating is much colder, and you get thermal grade of the order of about 100 degrees C between the hot and the cold surface. So all of this put together - this whole cooling technology effectively helps to keep the blade below its melting temperature.
Claire - So a combination of cooling channels and a thin ceramic coating helps to stop the blade from melting. But what about the blade material itself? Well, in such extreme conditions you need to use a special type of alloy, known as a superalloy. To find out a bit more about this intriguingly named material, I spoke to superalloy expert Professor Cathie Rae from the University of Cambridge...
Cathie - The turbine blades spinning inside the engine experience temperatures of over 1600 degrees centigrade, and the stresses the experience are equivalent to having a large truck hanging on every blade. Pure metals are not very strong. Think of a 24 carat gold ring - it wouldn't be strong enough to hold the diamond, so we add silver and copper to make it an alloy that makes it stronger.
The different sized atoms disrupt the regular layers making it more difficult for them to slide over one another. This is what happens when metals deform. Making alloys is rather like making a cake - you use the same ingredients but you can get very different results depending on the proportions you have. But it also matters how you combine them - think of crunchy biscuits or a soft sponge cake.
Superalloys are pretty unique alloys in that their strength increases or holds steady as they get hotter. They're made up of up to ten different elements, and though all of these are necessary to achieve the design properties, the essential ingredients are nickel, aluminium and chromium. Aluminium is a dream addition to nickel - it strengthens the alloy, it also gives it a protective coating preventing it from reacting with the oxygen, and it also reduces the density meaning that it stresses or lower as the blades rotate.
Claire - Superalloys, cooling channels, and ceramic coatings are key to keeping the blade cool but how exactly do they use these technologies to make a blade. The answer lies in a process known as 'investment casting' whereby molten alloy is poured into the mold in the shape of a blade. Interestingly, the mold also has some special internal sections which will eventually help to form the cooling channels as the alloy solidifies around them.
Back at Rolls Royce, I went into the casting section of the factory to see this process in action and I was accompanied by some very loud vacuum pumps...
When casting these nickel based superalloys, they have to be molten. So once the have solidified and the mold has been removed, the blades are heated up to about 1300 degrees celsius. A heating step evenly distributes the variety of elements within the blade and makes sure it has uniform properties. After this step, the blades go through a variety of inspections to make sure there are no problems. They then leave the factory where they continue their production journey. It is at this stage that the thermal barrier coatings are added to the surface.
To finish my visit, I went to see what happens when you put all of these blades together to make an engine...
I'm currently standing next to a Trent 700 engine, which is about... gosh 6 feet by 6 feet - it's pretty big. Now there is a lot of pressure on engine manufacturers to make engines more efficient. What is Rolls Royce doing to help meet these targets?
Neil - So one of the requirements to make an engine more efficients is to increase the turbine entry temperatures, which obviously means you now have to have allos which have got even higher temperature capability. So there's work that's looking at such kind of alloys, also looking at alternative materials like say ceramic matrix composites which are even higher melting which have have got much better temperature capability. But in all of this, it's not just important to have materials with high temperature capabilities but how can you manufacture them, and the manufacture capability of metals generally is a lot easier than making ceramics simply because metals are a lot tougher. So it's quite a challenging scenario and lots of work is being focuses on these alternative materials, both metallic and well as non-metallic.
Claire - So there's truly a lot more to these engines than initially meets the eye. And everyday researchers are hard at work investigating the next generation of high temperature materials. Through clever material science and engineering, we can keep raising the operating temperatures of these jet engines to improve their efficiency and produce sustainable air travel in the future.

38:07 - Flying close to the sun
Flying close to the sun
with Katie Hassell, Airbus Defence and Space
The Solar Orbiter, a joint project between NASA and the European Space Agency to study the Sun close up, is scheduled for launch in 2018. It will get nearer to the Sun than we've ever been before. Airbus Defence and Space were awarded the contract to build the Solar Orbiter, and so they have quite a challenge on their hands protecting it from the Sun's intense rays. Katie Hassell is a Senior Spacecraft Thermal Engineer with Airbus she explained to Connie Orbach just how close to the sun the probe will be going...
Katie - Solar Orbiter, as part of its mission, will going to a third of the distance from the Sun to the Earth, so the close third to the Sun - that's just inside Venus's orbit.
Connie - And what sort of temperatures is that then - that's a lot closer than we are?
Katie - Yes. So it has a really big heatshield on the front of the spacecraft and the front of that heatshield will see around 600 degrees. As an idea, if you've ever left a bar of chocolate in your pocket for a bit too long... it will do that to aluminium.
Connie - That's the front but the back must be quite a different temperature?
Katie - Yes, the back is cold. So the idea with the heatshield is that it works a little bit like a parasol in the sense it provides a shadow for the whole of the spacecraft and so, everything behind that heatshield is going to be cold. And it can see dark space which we model that around -270 degrees, so the spacecraft itself could get down to temperatures of about -180.
Connie - Wow!. That's a really huge range.
Katie - Yes.
Connie - And I'm guessing, if we've not got the atmosphere of the Earth, then I'm guessing there's a radiation issue as well?
Katie - So radiation is an issue, particularly for Solar Orbiter, because it's going so close to the Sun it does mean there's going to be a lot of charged particles in the environment that it's going to be in.
Connie - When you're thinking about these problems, this huge range in temperature, lots of radiation, how do you go about choosing the materials to make the Solar Orbiter from to deal with these conditions?
Katie - So we have a good idea of how certain materials behave. We know radiation's going to be an issue, which then starts to narrow down what type of materials we can use. So for Solar Orbiter, we're looking for anything that is electrically conductive, so anything that isn't electrically conductive we then basically remove that from the options - it's not available to us.
Connie - Why does it need to be electrically conductive?
Katie - That's these charged particles. We don't want static to build up on any part of the spacecraft because, otherwise, we could end up with electrostatic discharge; it's like little lightning across the whole spacecraft, and that can damage the instruments.
Connie - And so what about the other problems, the other materials?
Katie - So then, after that, it becomes quite a lot of a thermal issue. So what we're looking to do there is maintain the temperature of the spacecraft. Ideally, from a thermal point of view, it would be lovely if the spacecraft could be white. However, we couldn't find a white material that was going to maintain its whiteness during the lifetime of the mission so we're actually going to be using black, and by doing that it means that we can understand a lot more how the colour of the spacecraft is going to change over its lifetime. And the idea is we try to maintain a particular type of colour so that we're controlling the heat flow so from Sun, around the spacecraft, and then out to space.
Connie - So you kind of know what's going to happen right from the beginning as opposed to...?
Katie - That's the general idea.
Connie - OK. What are the materials then - what sort of things are you using?
Katie - Inside that shadow cone, we've got thermal blankets on there, they're a little bit like what you might see marathon runners being wrapped up in, but lots of layers of those. They're made out of aluminium foils and, again, they've got a black coating on the outside of those. And then the heatshield that I've already mentioned, that has a special paint on the very front of it that, it just so happens, is made out of burnt animal bones.
Connie - Ohh - that's quite surprising!
Katie - Kind of a random one!
Connie - Yes. There's a load of burnt animal bones going up into space?
Katie - Yes.
Connie - Why? Surely there's lots of things you could make which would do the job?
Katie - We've asked for a black paint that can meet all of our requirements and it just so happens that the black paint that has come back to us, that can maintain all of that electrostatic discharge, that can maintain it's colour, and isn't going to fall off once it's all painted on there, it's made out of charred animal bones.
Connie - OK so, basically, this kind of a big box and it's got this big square sunshield in front of it, which isn't made of aluminium - what's it made of?
Katie - It's made out of titanium.
Connie - OK. Made of titanium and covered in this black paint made of all things... crushed animal bones. So how do you know it's actually going to work?
Katie - Good question!. During the whole development of the mission we do a lot of analysis, so different teams do their own types of analysis. I'm a thermal engineer so I've been doing thermal analysis. And then what we do is we build it and we put it in what's called a solar simulation chamber and that is, essentially, a really big box that has a zena light bulb. And the xenon light bulb is to simulate all of the different wavelengths that the Sun emits its light in, and we put our spacecraft in there and we see how the spacecraft reacts to that sunlight.
Connie - So that xenon light bulb is like a mini-sun?
Katie - It's a mini-sun, yes.
Connie - Oh Wow! And that's just looking at it right in the here and now. I'm guessing you have to then do some sort of analysis on this?
Katie - Yes, we do more analysis at that point. So we make a model of the test chamber and what we do with our spacecraft model is we make sure that the temperatures on the model match what we're getting out of the test chamber, and then we run lots of simulations to model the different types of environments that the spacecraft's going to be in during its mission lifetime.
Connie - And how long is that mission lifetime - how long is it going out there for?
Katie - It works out at around nine years. It's got three years where it's actually going to get to the Sun, and then we've got three years where it's doing the science and then, all being well, it will have a further three years doing even more science.
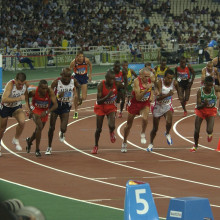
43:57 - Going for gold at 26 degrees Celsius
Going for gold at 26 degrees Celsius
with Dr Dan Gordon, Anglia Ruskin University
It's Olympic season but how are athletes from cooler countries going to cope with those Brazilian temperatures? It may be winter south of the equator, but temperatures are still predicted to reach 26 Celsius in the afternoons. How will this affect the, and what does the body do to keep cool? Dan Gordon is an exercise physiologist; he's also an athlete himself and has represented Great Britain in three different sports in the past and, as he explained to Chris Smith, he even holds a World Record...
Dan - The World Record is in track cycling; I still hold the outdoor World Record for a kilometer time trial.
Chris - So you must know all about handling the heat. So why is thermal situations a challenge for people out there in situations like this?
Dan - Yeah, it's an important question. The biggest challenge to the body's functioning is heat. If you think about muscle changing length and therefore generating force, 75% of the energy that's produced is lost in the form of heat. So we're producing vast amounts of heat and then we put someone into a hot environment, it really puts the body into a state of enormous compromise when you're trying to compete. And you're trying to generate very fast and rapid muscle contractions...
Chris - So it's not just dealing with having very good musculature and being highly trained, it's also being able to cope with the thermal stress of how to dump enough heat back into the environment to keep your body running efficiently in these situations?
Dan - Exactly, and that's the key to success. If you imagine you go to an olympic games and most of the athletes are of a fairly similar standard, if you take the top ten in the world they're a fairly similar standard. So one of the big factors that really determines the winner from the loser is the person who can cope with the heat and dissipate the heat the most effectively.
Chris - But can you train that, Dan?
Dan - You can. I mean you can train it in a number of ways. One of the ways is you can use what's called acclamation. So acclamation is where we put athletes or individuals into heat chambers and in the heat chamber you can expose them to the temperature you're going to get in the local environment, and the body will physically adapt. Or you can acclimatise somebody where you actually go to the hot environment and you're there for a number of days prior to whatever the competition's going to be.
Chris - And what sorts of changes or adaptations does the body make to your physiology in order to enable you to become more efficient at losing or controlling thermal stress?
Dan - OK, now this is the really cool bit!
Chris - Or not!
Dan - Yes, absolutely! If you go into a hot environment the first thing that actually happens is that you get an increase in the skin colour - the skin goes quite red, and the reason for that is the circulation is being redirected to the skin. The blood has a fantastic capability of dissipating heat from the body, so it's taking it from the core and dissipating it away from the body, so we get a redistribution of blood flow.
Chris - In most holidaymakers case that's just because of the local vino but we'll assume these athletes are not indulging...
Dan - In my case, it's just a natural sunburn.
Chris - So you bring the blood close to the surface of the skin and because the blood is hot, you've got a hot thing close to the skin so it's going to radiate some heat?
Dan - It's going to radiate the heat away from the body. But, that's quite an inefficient process because you're now redistributing the blood away and there's only a finite amount of blood and, of course, the blood carries the oxygen, and we have this compromise. So what we know...
Chris - It's not going to the muscles?
Dan - It's not going to muscles and it's being redistributed away from other organs. So what happens is, over time, as you start to expose something to the environment, and I think this is the bit that throws people, is you start to sweat more. We often think sweating is a bad response but, actually, it's a fantastic response because sweating is a far more efficient process in terms of that redistribution of blood flow, so the redistribution of blood flow is downregulated.
Chris - So you can train yourself to sweat more?
Dan - You can train yourself to sweat more...
Chris - How?
Dan - Well, you do it be exposing people to those hot conditions.
Chris - Do you get more sweat glands?
Dan - You don't get more sweat glands, it's just that what naturally happens is the body learns, through that exposure, to not get the redistribution of blood flow and then, therefore, promote the sweat response.
Chris - Ahh. So you're substituting sweating for rushing blood to the skin surface?
Dan - Correct.
Chris - So how much can someone sweat?
Dan - Well, I mean it really does depend. But you can see athletes loosing, I mean we're talking litres. But you also get individuals who don't sweat a lot. I mean there are a lot of athletes in Athens who we called 'dry sweaters.' They really didn't look like they were losing much but then you have a lot of athletes who sweat vast amounts, so it's very individual even in people who are acclimatised to the conditions.
Chris - And how long would it take - say you took me as a relatively untrained (at least in warm temperatures) individual and took me to Rio, what would you need to do to me to get me as acclimatised to cope with those temperatures and boost my sweat rate?
Dan - OK, so the key is making the most of the time. So in a normal situation, it takes about ten days to acclimatise to anything about 26 to 35/36 degrees C - about 10 days. We can speed that process up by doing acclimation prior to the event so, if we were doing acclamation every day for an hour to two hours a day, we could probably reduce the acclimatisation period down to about eight days.
Chris - OK. Now is there evidence that people who grow up in these countries - so if I am from Ethiopia I'm very good at marathon running not just because I've got good ways of extracting oxygen from my muscles, but because I've evolved to have very good, very strict temperature control - is that the case as well?
Dan - That is the case as well. So you know, unfortunately, the UK athletes had their time with London. We did well in London, it was a natural environment. We're now at a natural disadvantage and it's no doubt that the Kenyans, the Moroccans, the Ethiopians are at the advantage because they're got, as you say, great ability in terms of exercising and competing, but they've got that natural ability to dissipate heat and are really good sweaters.
Chris - Well they say a team always plays best at home don't they? It's certainly true in that case.
Dan - Very much.
Chris - I've always joked that the olympics is more a trial of genes and genetics than it is really about anything else. Would you agree with that? I mean it is basically your genetics against someone else's.
Dan - It is in the isn't it. It's no doubt that's what it comes down to and if you look at the bell curve, and we're right at the far extreme of the bell curve, and then at the far extreme of the bell curve, you're at the extreme of that extreme. And it really is about these extraordinary genetic specimens and, you know, it's a massive, massive component in terms of everything that's going on.
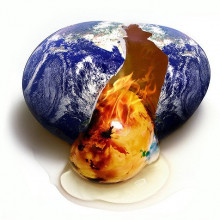
What would half the Earth look like?
Lucka Bibic asked Professor Marian Holness from University of Cambridge to find an answer to Robert's question...
If we can slice the Earth in half and leave the inner and outer core intact like cutting the avocado, what would it look like from space?
Lucka - For a glimpse of what Earth would look like from space, we turned to Earth expert Dr Marian Holness from the University of Cambridge.
Marian - Well the Earth is about 6,400 kilometres in radius. The crust, which is the bit we walk on and know most about ranges from about 10 kilometers thick to about 70 kilometres thick, so that's absolutely nothing really. So, if we go further down, we come to the mantle, which goes all the way down to 3,000 kilometres depth below the surface, and that's predominantly made of silicate minerals (magnesium, iron silicates), In the shallowest part of the mantle, that is dominated by a mineral called olivine and perhaps, paradoxically, if you look at all the text book pictures with that slice through the Earth, the mantle was always shown as red, presumably because it's hot, but actually the mineral olivine is green. So if it had cooled down, we'd see from space this great mass of green rock.
Lucka - Life underneath the Earth's mantle is actually green then. What can we see if we dig even deeper into the Earth's core?
Marian - If we go further down still, we get to the core and the core is made predominantly of iron and nickel. And since it's been cooling down ever since the Earth was formed, the centre of the core is actually solid now - it's been crystallised. So we've got this inner core that's solid and the outer core that's convecting.
Lucka - Alright then. But how did you figure out what is actually going on over 6,000 kilometres underground?
Marian - We can find out how heavy the Earth is and we know how big it is. So we can look at the rocks on the surface and we know what their density is, and we know that there must be something much, much denser in the centre of the Earth to make the Earth as heavy as it actually is for its size.
The next thing we do is look at meteorites that are coming to the Earth's surface and a lot of those meteorites that come are made purely of iron. So we can say, ah yes, we've got all these bits of iron coming down from the sky so it's likely that what we've got in the centre of our Earth is something very much like this.
Now how big is it? The question can be answered by listening to the Earth. So when the Earth experiences an earthquake, it rings like a bell and it sends seismic waves all the way through the Earth. And we can pick up those seismic waves using seismometers scattered all the way through the Earth, and we can tell how fast the waves are moving through the Earth and what type they are. So we can just piece it together from observations of meteorites and by listening to the Earth.
Lucka - So if we slice the Earth as we slice an avocado and see it from outer space, what would we see then?
Marian - We'd see this thinnest of thin skin, which is the crust, and then we'd see this mass of green mantle and then, in the centre, we'd get this beautiful, shiny, metallic core.
Lucka - Thanks Marian for explaining to us how Earth shows us its true colours!
Next week we will be answering Robert's question:
Is it possible to unlearn what we've already learnt?
Comments
Add a comment