Under the Microscope
Alongside analysing AI faces & how a heart beats, we will be putting microscopy under the microscope; from the first glimpses of life up close, to stepping inside the lens and experiencing microscopic specimens in virtual reality.
In this episode
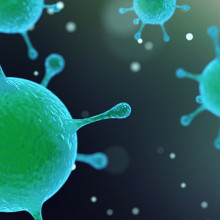
01:09 - Lassa Fever infections in the UK
Lassa Fever infections in the UK
Last week, the UK declared its first brush for more than a decade with a rare tropical disease, similar in some ways to Ebola, called Lassa Fever. An individual returned with the infection following a trip abroad and subsequently transmitted it to another family member. This also led to the infection of their newborn baby, which tragically died. Because the diagnosis was only confirmed later, there were many healthcare workers, at two hospitals, who had cared for the victims when they first became ill but may not have used the level of personal protective equipment required to defend against infections of this sort. As a result, a massive contact tracing effort has been launched to ensure that those staff members remain safe and to prevent any further onward transmission of the infection. The downside of doing this is that other healthcare activities have had to be limited owing to staff isolating. To tell us more about Lassa Fever and the history of its discovery, study, origins and impacts on our health, here are Julia Ravey and Harry Lewis…
Julia - Lassa Fever gets its name from the town in Nigeria, where the first cases were officially documented in January, 1969. A nurse Laura Wine, working in the Lassa Mission Hospital fell ill shortly after looking after a pregnant patient with what appeared to be the same disease. Both had a high fever and flu-like symptoms, severe fatigue, pains in the chest and muscles, and more alarmingly, bleeding from their gums. Sites where blood samples were drawn, continued to bleed afterwards.
Harry - Despite being airlifted to a bigger hospital, Laura Wine died the next day. Shortly after two other nurses who had been caring for her themselves became ill. And one of them also died. The other, Lilly Pinneo was flown to New York where she subsequently recovered in an isolation unit. The pattern of spread and the timing with which the cases developed symptoms suggests to the medical team that hitherto unknown virus might be behind the cases.
Julia - They sent the samples from Lily Pinneo and the other victims in Nigeria to a number of labs, including a specialist team at Yale University. Headed by Spanish doctor, Jordi Casals, who would set up a unit there to study, identify and classify exotic diseases.
Harry - Regrettably, and perhaps as a reminder of the power of the genie that they were trying to coax from its bottle, within three months, the virus nearly claimed its next victim. Casals' health developed the infection. Complaining of severe cold light symptoms and a running fever. He was placed into isolation and tests confirmed that he was positive for the very virus he was trying to study.
Julia - In an ironic twist of fate, having been the source of the virus that could kill him, the now convalescing Lily Pinneo then came to the rescue by allowing doctors to transfuse him with antibodies collected from her blood.
Harry - The intervention appeared to work. Once he recovered, together with colleague Sonya Buckley, Casals was able to resume work on the virus leading, within a year, to its successful isolation and identification, which they published together in the journal Nature. His own brush with Lassa Fever meant that Casals was in no doubt about the infectious and dangerous nature of this agent. His other major contribution was to lay the foundations of many of the biosafety protocols used today to safeguard against laboratory and hospital spread of infectious diseases.
Julia - Relying on these techniques, scientists have since been able to learn a lot more about Lassa Fever in the subsequent 50 years. We now know it's naturally carried by mouse species native to a number of countries in West Africa. Once the mouse picks up the virus, it becomes a lifelong carrier, shedding the infection in urine and faeces.
Harry - Humans exposed to this material, for instance when cleaning or sweeping, can inhale the infectious debris which can also make its way into cuts or other breaches in the skin. The symptoms usually take from a few days to a few weeks to kick in. Usually, a fever is the most characteristic feature.
Julia - A flu-like illness is common and because the virus attacks the linings of blood vessels, blood clotting can be affected, which is why some developed bleeding from their gums, back passage, and even sometimes their eyes.
Harry - Like many infections though, Lassa is more severe in pregnancy and often transmits to the developing baby; Usually lethally.
Julia - Surprisingly though, over 80% of the half a million cases that occur in endemic areas of West Africa each year are asymptomatic. About a fifth of cases are more severe, and about 5% of cases end up in hospital. The mortality rate is about 1 person in every 100.
Harry - The virus is present in blood and other body fluids, including urine, for several weeks. Men can continue to shed the Lassa virus in seminal fluid for several months after they recover, meaning that sexual transmission of this infection is well documented. People caring for Lassa Fever for patients, therefore need to take steps to avoid exposure to any of these body fluids.
Julia - We can test for Lassa Fever using PCR to detect the genetic material of the virus. Or we can look for antibodies to the virus in the blood. But there are no treatments for Lassa infection other than supportive therapy to keep patients hydrated and stable while they recover.
Harry - The antiviral drug ribavirin is sometimes used, but the evidence for its effects are quite weak. At the moment, there is no vaccine for Lassa, although experimental agents were developed in the past and appear to be effective. Perhaps, some are saying, the very useful RNA technology developed to help combat COVID 19 can be brought to bear against Lassa too, in the future.
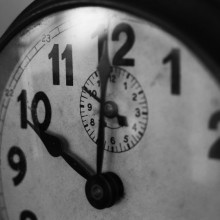
06:53 - Atomic clocks measuring time in millimetres
Atomic clocks measuring time in millimetres
Jun Ye, University of Colorado
CS Lewis once said “The future is something which everyone reaches at the rate of sixty minutes an hour.” But is that always true? While it certainly seems that time runs slowly whenever I’m in a boring meeting, physics tells us that in fact, the passage of time is not as constant as we once thought. Professor Jun Ye and his team at the University of Colorado are building incredibly precise atomic clocks to try to measure these distortions in time, as Robert Spencer found out...
Robert - In the 2014 movie Interstellar, the protagonists visit a planet deep in the gravitational pull of a black hole. Despite spending only a few hours on the surface, when they return to their colleague in a spacecraft, they find 2 decades have gone by.
Jun - People find that hard to believe; That time is all relative. There's no absolute time.
Robert - It's called time dilation and it all depends on where you're standing. The key is gravity.
Jun - Space and time are interconnected. When we get close to a massive body, both space and time will be curved.
Robert - That massive body could be a planet or a star. Curved space causes objects to fall towards the body, like a ball falling to the ground or a spacecraft into a black hole. The curvature of time is more mind boggling.
Jun - As you approach a massive body, the time that you measure will slow down.
Robert - It's all the results of Einstein's theory of general relativity. But it's not just a bunch of equations on a Blackboard or a plot point for sci-fi.
Jun - This was a theory, however it's being tested over time and is found to be consistent with experimental findings.
Robert - To test this theory, all you need is to take a clock to a place with weaker gravity and one to a place with stronger gravity and compare how fast they tick. For example, objects in orbit will clock up a different amount of time to us on the surface of the earth.
Jun - And the atomic clocks on board of satellites need to take into account of this time dilation effect.
Robert - In fact, if they didn't GPS would stop working in a matter of hours. But we don't need to go to a black hole or even into space at all to measure this effect. If I put a clock upstairs in my living room and one downstairs in my bedroom, there's a difference.
Jun - A clock downstairs will tick slower than a clock upstairs because downstairs is closer to the center off the earth.
Robert - And that's why I was late to work this morning. My alarm was delayed due to relativistic effects.
Jun - That's not necessarily true.
Robert - No, perhaps not. The effect would be so tiny that we wouldn't be able to detect it. Or could we? Jun Yee has been building the world's most precise clocks and he's gotten pretty good at it. Unlike clocks we may think of, which use the ticking of a pendulum to measure time, Ye's clocks use the ticking of electrons around atoms.
Jun - The atom we use is called a strontium atom.
Robert - They use lasers, shooting, very precise photons at these atoms to interrogate them.
Jun - We can use the frequency of the photon as a handle to tell the time.
Robert - And with high accuracy in measuring time, comes the ability to measure the slightest of changes in gravity. In fact, rather than needing the tens of hundreds of miles to satellites or the few yards to upstairs, Ye's team can measure the difference in gravity between two clocks separated by...
Jun - Just a mere millimeter.
Robert - Yes, that's right. 1 millimeter. The difference in the speed of time here is close to nothing. Each second, the lower clock loses.
Jun - 0.0 0 0 0 0 0 0 0 0 0 0 0 0 0 0 0 0 0 1 seconds.
Robert - That's 19 zeros.
Jun - This is incredibly precise.
Robert - But Jun Ye and his team are not satisfied with simply measuring gravity and time to this level of accuracy. By measuring the curving of space itself, he hopes to build tools with very real applications.
Jun - You can turn that into geological survey tools to sense the change in Earth.
Robert - By measuring how the mass moving underground distorts time itself. He hopes to be able to predict volcanic eruptions or measure glacier's melting. Or perhaps we could measure changes in gravity not caused by normal mass.
Jun - We may be able to shed light on the mysterious matter called dark matter. It's in our universe, but has eluded our detection.
Robert - Perhaps the most incredible is that when you start measuring gravity on such small scales, you start to probe the relationship between general relativity and quantum mechanics.
Jun - Quantum mechanics describes the microscopic part of the world. How atoms, photons & electrons evolve. General relativity is typically associated with the macroscopic view of the world.
Robert - Getting these two theories to play along together has left physicists scratching their heads for decades. Now measuring the intersection of these models seems within reach.
Jun - It will be fantastic if we can start to connect the very microscopic world of quantum mechanics with the very macroscopic world of general relativity.
Robert - The gravity of this discovery cannot be understated. It may bend our understanding of atoms, space, and time itself. Or perhaps it'll just confirm what we thought to higher and higher precision, or something in between. It's all relative.
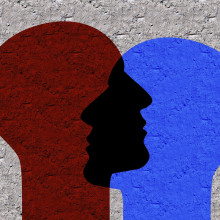
12:49 - Fake AI faces are deemed trustworthy
Fake AI faces are deemed trustworthy
Sophie Nightingale, University of Lancaster
We have entered into a new era of technological advancement. Mechanical evolution may appear to have slowed down to the likes of you and I, but in the world of computer science there’s an unprecedented increase in novel developments. Part of this is down to the computing communities' open access ethos, domains like GitHub allow users to share, tweak and comment on new softwares. But there is a dark side to this genius, as Sophie Nightingale from the University of Lancaster explains to Harry Lewis. They start by looking at a website you can visit too, it’s called thispersondoesnotexist.com...
Harry - You are telling me - this is genuinely true - that this person doesn't exist. I'm confronted by a Caucasian, blonde female, and she looks maybe in her mid twenties, and she looks completely real, Sophie. I can't fault this at all. It looks like it's straight off Facebook or something like that; a LinkedIn profile, maybe.
Sophie - Absolutely. That is somebody who does not exist in the world.
Harry - Okay. Let's break this down: we are talking about these being synthesized by a type of algorithm by artificial intelligence. What does that consist of?
Sophie - This is a type of machine learning. It's a relatively new type known as generative adversarial networks or GANs. What's quite special about these is they use two neural networks which are pitted against each other. So, imagine a two-player game where you're in battle with your opponent: one of those networks is a generator the other is a discriminator. The discriminator is given a large collection or corpus of real images, and in this case we're talking about images of faces, of people who are real. Then, the generator's task is to try and synthesize an image that's good enough that it manages to trick the discriminator into believing it's a real face. Over time, it receives feedback from the discriminator and it will refine its parameters and eventually generate a face that the discriminator can't actually tell apart from those real images anymore.
Harry - So, if you were to start this off: you write the algorithm, you give it the information it needs to begin, and then you step back and you leave these two networks to themselves - there's no longer any human interaction.
Sophie - Absolutely, yes. This is what's known as unsupervised machine learning. There's no need for a human to do anything once you've given it the original corpus of images.
Harry - And in your research, you found that the average person trusts these synthesised faces, sometimes more than images of real life people?
Sophie - Yes. On average, we found that people's ratings of the synthetic faces were slightly higher than the real faces. Now, it wasn't a huge difference, but it was significant.
Harry - Why is that so exciting? And why is it also quite terrifying?
Sophie - This is an incredible advance in terms of technological capability and there's definitely potential to use these for good. For example, we can use and apply these to security and defence systems, but there's also the flip side of making this technology accessible to everybody and sharing it openly, which means that there's a lot of potential for harm as well: for revenge porn, financial fraud, adding to disinformation and misinformation on social media, and many other novel ways that perhaps we are not even yet aware of. And the other thing is the liar's dividend: it allows for any unwelcome recording that is in the media to be denied by somebody. They can simply just call in to question its authenticity.
Harry - I get the impression that what you're alluding to might be the future of where this technology goes. It's not just perhaps limited to pictures in the future, it could go into sources of video content and audio content?
Sophie - Absolutely. That's exactly right. I would say we're not far off.
Harry - And looking to the future with your research, I get the impression it's sort of a call to arms.
Sophie - If nothing else, the main thing I wanted to get across is that we need to do something about this. You could build and embed watermarks into images and video synthesis networks so that, down the line, when these come into play, we can check and actually have a way of reliably identifying if it's a synthetic image or video. It's important to do this now because it's likely that other forms of AI synthesised content, for example audio and video, are on the path to being indistinguishable from real content as well. Once that technology is released, we can't take it back. Once it's in the world, we can't then put it back into a box.
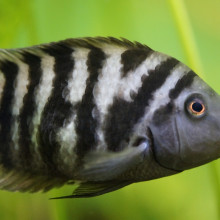
18:09 - Robofish powered by heart cells
Robofish powered by heart cells
Kit Parker, Harvard University
Studying how the human body works is no mean feat. And trying to figure out how the heart - our automatically-beating, life-dependent organ - functions requires scientists to think outside-of-the-box. Julia spoke to Kit Parker from Harvard University, who is making waves with his novel approach to understand how our heart cells operate…
Julia - Almost every second we're alive, our heart beats. This process is automatic, orchestrated by the cardiac cells which make up our blood pumping organ. Kit studies how these cells work and came up with a somewhat unconventional way of doing so.
Kit - Morphologically, it looks like a fish. Functionally, it looks like a fish. Genetically, it's human.
Julia - He got his inspiration to do this from a trip with his daughter.
Kit - In the New England aquarium of Boston, there is a display of jellyfish. I was watching these things swimming around and I was thinking, "that thing's pumping just like the ventricle in the heart" and said, "I bet I could build that."
Julia - Using heart cells, Kit wanted to see if he could mimic the pumping of these marine organisms to learn about how the heart functions.
Kit - So we built a jellyfish - and that was quite a scene because we took a rat apart and we rebuilt it as a jellyfish. It was alive and swimming around.
Julia - Next up on Kit's list (after another aquarium trip), a stingray.
Kit - I said, "I bet I can build that one too." You know, now my daughter's always teasing me: "Oh you gonna build that Daddy." Whenever I see something cool: "You gonna build that. You think you can build that."
Julia - Why Kit is keen to use heart cells to build constructs in the lab is to improve regenerative medicine: a field which aims to build human hearts and other organs in the lab to give to people who need them. But a lot of focus in this area has been elsewhere.
Kit - Mostly, it boils down to trying to replicate the anatomy, but there's some pretty complex physics there in the heart. We decided to pick a couple principles of cardiac biophysics and build a very simple model: the fish - and we chose the zebra fish.
Julia - Kit and his team made a pacemaker: a bundle of cells like those in our own heart to alter how fast or slow it beats. This pacemaker is called a genode. They then constructed two parallel layers of heart cells derived from human stem cells, embedded in gelatin, with a fin across them to balance, and set them off beating.
Kit - And if you give a little bit of a tickle to the genome, it sends a wave of excitation through the tissue and that's what causes it to contract.
Julia - When the pacemaker kicks in, the heart cells in one layer contract, stretching the other layer over to the side, which then prompts it to contract, creating this swishing back and forth like a fish's tail, which allows it to swim. And they could keep this up for a while.
Kit - They would swim for weeks on end - for about four and a half months they would swim with the same velocity. This is a big advance, Julia, because when you're born, the number of cells you have in your heart, two days after you leave the womb, is the same for the rest of your life unless you have a heart attack and some of them die. Those cells have to rebuild themselves as they grow and as they pump.
Julia - It's thought human heart cells rebuild themselves every 20 days.
Kit - With our fish that were pumping for 180 days, that means they rebuilt themselves five times. That's a big deal.
Julia - Multiple healthy rebuilds while the cells are functioning is a great sign for Kit's overall goal.
Kit - Eventually, what we want to do is build a heart for a child that's born with a malformed heart. Building with biophysics, understanding those design criteria, and the longevity of the tissues that we build, are two big, important steps for us. We think we learned a lot from that.
Julia - But, sadly, the human fish are no more. They were a lot of work for Kit and his team.
Kit - It's like having a child, right? You've got to feed it, change it's diaper every day. It's kind of the same thing with these fish. You've got to come into the lab every day and so after a while you're just like "This thing's never going to stop." People are always disappointed when I tell them. They say, "Well, what are you going to do with the fish now?" I'm like, "Nothing it's over. We're never going to build another one." It was a training exercise to see how good we were at building human cardiac tissue. Before I can build a heart that goes into a sick child (because it's game on when that happens - there's no turning back) I have to continually test myself to see how good of an engineer I am. So, with these marine organisms, by going to more and more complex anatomies, we test ourselves as engineers.
Julia - This is a pretty new field of science.
Kit - It's a cross between marine zoology and robotics. So, maybe 'zoobotics' is the word for it, I don't know.
Julia - By using this technique to build more complex marine creatures, this can hopefully tell us more about how our own hearts work.
Kit - The idea of using robotic design as a scientific instrument, the same way we use a microscope, is a really cool concept to me.
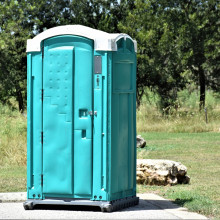
23:19 - Parasites, Poos and Portable Loos
Parasites, Poos and Portable Loos
Sophie Rabinow
Washing our hands has become paramount during the pandemic, but the Romans didn't seem to bestow any importance to this, resulting in parasitic infections. Anoushka Handa spoke to Sophie Rabinow to tell us more...
Anoushka - I'm glad I've never had to think about whether a pot has remnants of poo in it until now. Conical pots found near public latrines were previously thought to be storage pots, and have actually turned out to be chamber pots from the fifth century: portaloos for ancient festivals. Archaeologists at the University of Cambridge have found parasite eggs known as whipworms within the crusty materials residing in these old chamber pots from 1500 years ago. This makes me think, will the disgusting portable loos that we find at festivals be investigated in 1500 years time? I really hope not. But how did these parasites survive for so long? And what do they look like?
Sophie - The eggs are protected by a tough external shell, which is similar in composition to the exoskeleton of insects. The sample initially looked like a bit of grey dust.
Anoushka - These were analysed by the theme this week: microscopes. The researchers used a microscope and found eight parasitic eggs, which concluded that this part was indeed a chamber pot. I mean, that's a bit disgusting knowing that what you've put some stuff in has had faecal matter in there before, and I would be really resistant to working with poo. So how did archaeologists get over this hump?
Sophie - Archaeologists find faecal matter in general fascinating because it carries so much information about people in the past. These particular samples were quite nice, but I have to admit that some of my other samples in the past have been quite moist and smelly.
Anoushka - Poo and wee isn't something you'd want to think about on a regular basis, and the Romans didn't seem to either. Talk about pooing where you eat.
Sophie - The whipworm infections may have been acquired from the Romans eating with their fingers or from them eating fruits or veggies that were poorly washed and may have been manured with poorly composted faeces.

26:49 - The origins of microscopy
The origins of microscopy
Sietske Fransen, Max Planck Institute for Art History & Keith Moore, Royal Society
We are going to be getting up close and personal with our own cells by exploring microscopy. And not only are we looking down the microscope, we are getting inside our specimens. Anoushka Handa spent the week walking around inside a human immune cell. It started 350 years ago. Sietske Fransen from the Max Planck Institute for Art History and Keith Moore from the Royal Society gave an insight into the man who had the first glimpses of life under the microscope, Anthony Van Leeuwenhoek...
Anoushka - Not only are we looking down the microscope, we're getting inside our own specimens.
Julia - You what?!
Anoushka - I've spent the week walking around inside a human immune cell.
Julia - I'm sorry that doesn't really help me. What are you on about? How?
Anoushka - It's been a bit of a journey and it started around 350 years ago. Sietske Fransen from the Max Planck Institute for Art History and Keith Moore from the Royal Society, gave me an insight into the man who had the first glimpses of life under the microscope, Anthony Van Leeuwenhoek.
Julia - I am very excited to hear about what he saw.
Sietske - There is a possible link with his profession. He was a cloth merchant. And cloths merchants used magnifying glasses to look at the cloth they were buying and selling because they could count the number of threads per square centimetre. That would say something about the quality of the cloth that they were trying to buy and sell. Maybe his interest came out of his day job, but at the same time, he was definitely doing science for what he would have described as doing science. And I'm saying that because he was really keen on writing his experiences or describing his experiences to the fellows of the Royal Society. And he wanted to be part of that crowd. People often say about Anthony Van Leeuwenhoek that he was secretive and he was secretive about one thing. And that is how he made his microscopes.
Anoushka - What was his microscope? How did he make it? What does it look like? How big is it?
Sietske - Nothing you would think of if you now think of your binocular microscope that you might have used at school. It's a very tiny instrument. It's about four or five centimetres in height. There are two metal plates in between which there is a small glass ball, which is the lens.
Anoushka - What kind of specimens did he look at?
Sietske - He looked at everything. So the first experiments that Leeuwenhoek is sending to the Royal Society are of a bee or of a louse. But very soon after he also starts to look at bodily fluids. So blood, sperm, saliva, he takes a lot of his things that are immediately on him or around him because he also looks at his own hair. All tiny things that he can put on that microscope.
Anoushka - When were they found? And have they been replicated since?
Sietske - We know that he sent whole sets of them to the Royal society and they've gone missing. The ones that have been found, some of them are already no longer seen as original microscopes and others have more recently been discovered. It's only discovered in the past few years how we actually made the lenses because of course, of the few microscopes we have have left, they're made with dropped glass. So, it's a little glass ball and the melting of glass and making a small drop is not hard. However, Leeuwenhoek was grinding them. He was a showman. I think also he saw it as a case of pride to be accepted by people like the fellows of the Royal Society.
Anoushka - What better way to understand Leeuwenhoek than to go to the Royal Society to see if I could have a look at these letters myself. I met up with Keith Moore who brought out four massive volumes of letters.
Keith - We can start at the very beginning here. There are drawings in the margins sometimes. So they're all coming from Delft. You can see the date on this one very clearly 7th April 1674. So this is the beginning of his series of researches sent to the Royal Society. What you get here are not just textual descriptions of what's happening, but also drawings. And he sent them over so that the observation could be seen as a visual resources in addition to being a textual one. And he also at certain point sent over packets of specimens, so that fellows of the Royal Society in their meetings could try and replicate the observation.
Anoushka - I've heard that he's looked at his own blood, semen, and sweat. Did he send those over?
Keith - No, happily not. I mean, he used his own body as a means of finding things to look at under the microscope. It must have been an absolutely wonderful time to be a scientist. This was because almost whatever you looked at under the microscope would be something new, something that no one had seen before and therefore Leeuwenhoek and his whole generation of microscopists were really pushing a new frontier.
Anoushka - So let's have a look at some of the images. This is dated...
Keith - January 1680 from Delft and it's to Robert Hook because by this time he's the secretary of the Royal Society and Leeuwenhoek is sending over very beautiful drawings to him. He wouldn't have done them himself, so Leeuwenhoek, would have handed his microscope over to an artist and it was quite collaborative.
Anoushka - What else are we seeing on this page here? So we can see a centipede...
Keith - That's right. Centipede or a milipede. You've got a fly here, got some grubs as there are. Leeuwenhoek's letters very often send multiple observations. He'll incorporates several different things into a letter.
Anoushka - So he's just going out into the world with this microscope in his pocket.
Keith - That's absolutely right. What is this thing made of? How does it work? What's it in terms of living creatures? What are the structures that make up this thing?
Anoushka - Can I have a flick through these books?
Keith - You can. Leeuwenhoek did develop other kinds of microscope, things like water microscopes. So he could look at things that were living.
Anoushka - These are seeing things on around a millimeter scale?
Keith - It depended on the type of microscope Leeuwenhoek was using. He manufactured different types of lenses depending upon what he wanted to look at. So there are very high resolution instruments. If you're getting down to looking at bacteria, that is high resolution. But for most workaday things, he could use a lesser resolution. So he was using microscopes that were designed and made for a particular purpose.
Anoushka - Is that a whole fish?
Keith - That's a whole fish. So that's presumably quite a small one, but he's looking at some of the structural elements as well. There we go. One rats testicle.
Anoushka - But it looks like there's loads of swells here and waves. I'm guessing that's just the tissue?
Keith - That's right. So you're just seeing tissue floating in liquid there.
Anoushka - So Leeuwenhoek goes from looking at a whole fish all the way down to bacteria. Not only has he crafted lenses for different kinds of microscopy, but he can look through that whole range.
Keith - He was a remarkable individual and not just a good scientist and observer, but a remarkable art designer for being able to construct things like that, in order to observe such a range of material,
Julia - Sounds like you had an absolute ball!
Anoushka - It was teste-ing that's for sure.
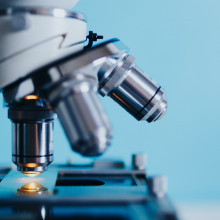
34:21 - 3D-printed microscopes changing fieldwork
3D-printed microscopes changing fieldwork
Richard Bowman, University of Bath
You can now 3D print microscopes. Anoushka Handa & Julia Ravey spoke to Dr. Richard Bowman from the University of Bath to tell us a little more about how they work...
Julia - It seems Leeuwenhoek's microscopes are much smaller than those you'd see in a biology lab. Are such small microscopes still useful today?
Anoushka - Actually, yes. Now you can 3D print microscopes. We have Dr. Richard Bowman from the University of Bath on the line to tell us a little more.
Julia - Richard, 3D-printed microscopes are not what comes to mind when I think about getting a closer look at samples. How did you design these microscopes and what have you been using them for?
Richard - Thanks. Well, there are quite a lot of nice ways to make a very simple, but very powerful microscope. For example, taking a webcam apart. But often the really hard part is the mechanics. And it's exactly the same challenge that Leeuwenhoek would have had, which is that you've got to point the microscope at the right bit of your sample and then bring it into focus nicely. Big microscopes do this with lots of very nicely made sliding mechanisms. One Friday afternoon in the lab, I was curious how much of that you could do with a 3D printer. And it turns out that if you use bending of the plastic, rather than sliding, you can build, more or less, all of the mechanics of a pretty capable microscope with a single 3D printed part. It started as a bit of fun, but actually it turns out it's useful in lots of places - if you want to put a microscope in an incubator or a fume hood, or even if you want to take a microscope to places where they're very difficult to get hold of. And so a lot of our work is with colleagues in Tanzania, where we're hoping to make them the first commercial producer of microscopes for medical diagnostics in the country.
Julia - I was going to ask, why would you 3D print a small microscope rather than use a microscope typically seen in the lab. Are these microscopes are used widely outside of a laboratory setting?
Richard - Yes, they're used all over the place. And, because we released it as an open source hardware design, actually, I don't know where they all are, which is a large part of the fun of it. We recently clocked them in over 30 countries from a brief survey of our forum. And they're used everywhere from community groups to university research labs. We are evaluating them for medical uses at the moment, although I should say they've not quite achieved the certification necessary to actually use them on patients yet.
Julia - Microscopes popping up all over the shop there. But a 3d printer is not that easy to come by, not something you see every day. So how accessible are these microscopes with the areas that they've been designed to be used for?
Richard - I mean, I was really surprised. We've been working with our Tanzanian colleagues for about five years now and actually, their business started out selling 3d printers. And they were locally manufactured, often made with locally available materials, and ideally quite a lot of recycled parts from e-waste. So , they didn't look exactly like the printer that was in my lab, but they could get hold of them. And actually if you compare the cost of a typical 3d printer with the cost of a typical lab microscope, you only have to print one before you've broken even.
Julia - That is super interesting. And it's amazing how you're both using technology and also, it sounds like, sustainability as well, but what is your big hope for the future with these 3D-printed microscopes?
Richard - I think the real aim of the project is about reproducibility and accessibility of this kind of science. So, I would love for labs that are currently priced out of being able to do exciting microscopy, whether that's in hospital settings or in research settings to be able to enter there. And not just to sort of accept what we've put out there, but actually to take ownership of the project and do something exciting with it. And in the same way, it's really great to see people use the open flexture microscope as a platform to build more exciting instruments on top of. And what I hope is that pushing this approach will get us back to the way that science was often done, where we would replicate each other's experiments, which is often very hard to do these days.
Julia - Brilliant. Well, we'll definitely be looking out for the microscopes and their use in the future. Thank you so much there to Richard Bowman.
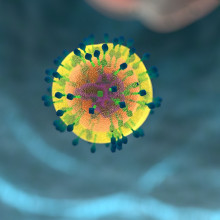
38:45 - Lights, lasers and microscopes
Lights, lasers and microscopes
Ed Sanders, University of Cambridge
Microscopes can be used to see finer and finer details of biological specimens such as T-cells. These are about 10 microns in size - which is about 10 times smaller than the width of a human hair. Ed Sanders from the Lee Lab at the University of Cambridge walked Anoushka Handa through these imaging techniques...
Julia - I can't believe you can print a microscope, but surely we need other instruments to let us really see the details of say, our own cells.
Anoushka - Well, there are different types of microscopes that let us see finer and finer details of small specimens like human cells.
Julia - How big was that immune cell? You said you were supposedly standing in?
Anoushka - That immune cell called a T-cell was about 10 microns in size. Now that's about 10 times smaller than the width of a human hair. And the proteins on that cell are even smaller than that.
Julia - So how do you look at them?
Anoushka - Well, using light lasers, tags, and electrons to zoom in further and further on these pretty small cells, Ed Sanders from the Lee Lab at the university of Cambridge, walked me through these imaging techniques.
Julia - Anoushka you didn't tell me we were gonna play laser tag.
Anoushka - I've headed over to the Department of Chemistry to have a look at a human T-cell, under a microscope. T-cells are a set of white blood cells that are part of the immune system. They're activated when fighting infections and foreign particles. You can imagine them as a bodyguard of sorts, which comes out when those cold or COVID particles come and attack.
Ed - It's quite important to know how T-cells make these decisions and how the decisions downstream would lead to either someone fighting off a disease or in the case of vaccines, how the vaccine would work is all due to your T-cells recognising something and then priming itself for a later infection. We know that T-cell triggering would lead to the immune response. Those very initial stages are actually very poorly understood. And partially this is because of the lack of tools to look at them properly.
Anoushka - Now, there are certain tools that we can use to help us understand the initial stages, and microscopy bears a large burden of this. There are various kinds of microscopy, electron microscopy and fluorescence microscopy, but how do they work? And which would be best to use in this case?
Ed - Electron microscopy would look at a difference in electron density. Whereas fluorescence microscopy would look at a molecule tagged with something that glows and you can use the glow to say where things are. So we could look at how certain molecules move on a T-cell surface with fluorescence microscopy. Whereas you could not do that with electron microscopy.
Anoushka - We're gonna be having a look at some human T cells.
Ed - First we're gonna use normal white light microscopy. So this is what you'd see in a typical biology lab in schools. And essentially what you'll see is a spherical blob.
Anoushka - Is that a technical term?
Ed - No, it's not a technical term at all, but it's the best way to describe what you're going to see.
Anoushka - Wow, that's incredible. These are human T-cells that I can see under the microscope. And what I can see is like Ed said these spherical blobs, but I can also see these little lines coming out of these spherical blobs. What are they?
Ed - Those are microvilli. These are long fingers that the T-cell will use to help it scan for a pathogen.
Anoushka - So these fingers I can faintly see them. Can we just use electron microscopy to have a look at the structure of them?
Ed - Well, electron microscopy would do that very well, but they can't necessarily look at the single molecules that are involved when the T-cell would make a decision of, whether something is a foreign invader or whether it's something that's meant to be there in your body.
Anoushka - Now, this is where a type of fluorescence microscopy comes in called super resolution microscopy. Super resolution microscopy is a type of fluorescence microscopy technique. You sacrifice temporal information, time for spatial information, space. You can have a look at anything to be honest. So long as you can tag it with a fluorescent dye, we're able to stick it on our microscope, illuminate it with our laser, and a subset of these fluorescent dyes are turned on at different points in time. And by doing so, we create this image of a bunch of different points, which we can then collate together and get our data from. And that way, not only are you passing the diffraction limit of light, which is 250 nanometers, you're gaining information that's already there, just couldn't be seen in normal fluorescence microscopy. But what processes happen on this scale?
Ed - The T-cell example is actually one of those processes, a single T-cell receptor and the interaction between that and an antigen can lead to downstream signaling. So we need to be able to get down to single molecule scales, where we can actually see what's happening on that level.
Anoushka - How do you stop fluorescent molecules from turning on at the same time?
Ed - So there's a few ways to do this. You can either use molecules that are photoactivatable, so we'll only turn on when exposed to UV light. You can use chemistry to help the molecules turn on and off. Or you can have a probe in solution that will bind and unbind. These three techniques are essentially the painter's palette for super resolution microscopy.
Anoushka - You've kindly labeled a T-cell with a fluorescent molecule. And what are we actually gonna look at when you image?
Ed - So today we're actually going to look at the membrane of the T-cell. These fingers actually play quite a big role in T-cell signaling. So we need to be able to accurately identify the structures. When I turn the lasers on, what you'll see is you'll see little flashes of light on the camera. So spots and those spots are single molecules in your sample, turning on. That's literally a single molecule that you're seeing there glow.
Anoushka - Incredible. We're seeing this in real time. How long does it take to actually get an image or a structure of the cell?
Ed - It'll take hours, because every time that we look at a single molecule and decide where that is, essentially, we need to build up the full picture of the T-cell. We need lots of molecules to turn on and build up this pointillist image.
Anoushka - Can we look at any other proteins on the cell at the same time?
Ed - For sure. So with the cell membrane, what I've done is I've used a probe that will bind the cell membrane in and then leave and bind on and off. If you wanted to look at a protein, you can use an antibody to bind that protein, and again, you'd have your fluorescent molecule there. All you really need for super-res is a way to attach a fluorescent molecule to a target of interest. And then secondly, somewhere to make those molecules blink.
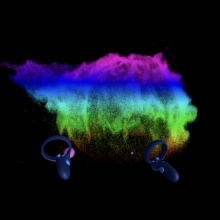
45:48 - Immersing Data into Virtual Reality
Immersing Data into Virtual Reality
Alex Spark & Alex Kitching, LUME
Anoushka Handa spoke to Alex Spark and Alex Kitching, the co-founders of a nanotech company, called LUME, to demonstrate their new use of Virtual Reality to view super-resolution micoscopy data...
Anoushka - I met up with the founders of LUME, a VR company that's based in London to have a look at the human T-cell that Ed and I had imaged using a VR headset. LUME is a nano imaging software company, specifically working on super resolution microscopy and the visualization of 3D super resolution microscopy data. But what is virtual reality?
Alex S - VR is where you're in a completely virtual environment. And you interact with everything virtually. You have two screens in front of your eyes, which gives you 3D stereoscopic vision and projected onto that as a completely digital environment.
Alex K - Instead of playing a video game in the traditional sense where you have a controller and you're looking at your character through a TV screen, you are actually in the video game. So when you look right your character is looking right.
Anoushka - Can I step into this human T-cell that was imaged for me earlier this week,
Alex S - Step right this way,
Anoushka - Right, I'm gonna put the headset on. That's on, where are the controllers. Okay. I think I've got them. So what do I have to do first?
Alex S - One controller goes in your left hand, the other one, your right. They have some tactile thumb feedback and triggers on the back. So it's sort of like an Xbox controller, but split in half.
Anoushka - I'll load the cell up. I'm guessing, menu. And then this cell?
Alex S - Yeah. Load data. And then yeah, click that cell there.
Anoushka - Oh, wow. That was really quick. That took like a couple of seconds to get up. And there's about a million localizations in this one. What can I do in this zone now?
Alex S - First thing to always do is just move around it, check it out. We've done a lot of work to make sure there's absolutely no motion sickness you'll feel with VR. So you have complete smooth movement.
Anoushka - I'm gonna try and walk through this cell. This is incredible. I can see the fingers like Ed was telling me these microvilli that are coming out the cell and it looks absolutely beautiful.
Alex S - So you can grab, move the cell around, rotate your way around it. You can color the cell by any dimension you want, not just X, Y, and Z dimensions, but also intensity or frames. And you can also get into the more analytical parts and you can select, for instance, the fingers of the data. And then you can go into the scripts and then run the certain analytical scripts on those areas that you selected.
Anoushka - I'll try and capture this single finger. And how easy is that? Because if I'm doing that on a screen, it's relatively difficult to get a single finger.
Alex S - Why don't you have a try?
Anoushka - Okay. Right. I'm gonna navigate my way on this menu and see if I can figure this out. I think I've got it. Cool. Okay. And now can I analyze just the isolated data?
Alex S - Yes. And it'll be super fast as well.
Anoushka - There's so much I can do with this. There's visualization, segmentation, exploration and analysis. Those are the four. So I can basically hold this cell in the palm of my hand as well as stand well in the midst of it.
Alex S - And I think what's interesting is because you're in a virtual reality environment, you can make this data as small or as large as you please.
Anoushka - That's wonderful. So you can literally just throw around the cell that we imaged... Bit dangerous.
Alex S - Yeah you don't want to throw around valuable data.
Anoushka - How did you guys get into using VR as a visualization technique?
Alex K - We met with Professor Steven Lee at the science museum where he was presenting their work in super resolution microscopy. We saw their work, and thought it was quite underwhelming to just look at these massive three-dimensional point cloud structures of cells, just using posters. We thought we could actually help with our VR experience to bring those to life a little bit.
Anoushka - What can it show that a screen can't?
Alex S - If you have three dimensional data, then looking on a 2d screen where you can end up with an obscurance so you could have data overlaying data, so you can't see behind it. Whereas in VR you'd be able to see that because you'll have two slightly different perspectives and you'd be able to move around it so you can get a rotation around it so that you could perceive its three dimensional nature.
Anoushka - How can we use virtual reality to our scientific advantage?
Alex K - I think you can use it in two ways. In our case, we used it very much to try and reveal hidden insights within the data. So by analyzing it and using the VR as a medium to really dig deeper into the data. But I think another approach is also to bring people closer together, to collaborate around and over this incredibly complex data. And I think there's a plethora of different applications from education collaboration, and just VR is a great medium for, or those connections to happen in a digital way.
Anoushka - I found it pretty amazing to try the VR kit on and to walk around this incredible cell, but I'm always a little skeptical of VR. Is it just a buzzword or people actually become immersed into the concept?
Alex S - I think there are definitely some people on the warpath to VR. I mean famously Mark Zuckerberg, he did a press release internally to his company in 2015 that said we're gonna completely transition the whole company and everything's gonna be in virtual reality by say 2025.
Alex K - The world that maybe is portrayed by Mark Zuckerberg of like wearing the VR the time. I don't think we're quite just there yet.
Anoushka - But Apple are now bringing out a VR headset later this year I think. Do you think that that will have a big selling point for the general public?
Alex K - It's the first step towards widespread adoption. It's going to be great for us and everyone who already has a presence in that space.
Julia - Okay. I get the boasting now that is incredibly cool.
Anoushka - I know!
Julia - What were those four things that you could do?
Anoushka - So the four things were visualization. So you can look at the data, you can walk around the data, you can have a look around it and share that experience with someone else. Exploration, which is again, walking in the cell, playing around with the cell, making it bigger, making it smaller, in the palm of your hand, throwing it around. Not that much though. Segmentation, so you are cropping, maybe specific fingers that Ed had mentioned, from the cell membrane and then finally analysis, where you are analyzing those fingers, the isolated data, or the whole cell as well. And you can do that with a various number of scripts.
Julia - And you were obviously standing still in like a static image of a cell. Do you think in the future, there could be a way that we could be in a cell that's sort of live and in action and moving and we can look at the cell as it's functioning.
Anoushka - Well, possibly. I think what we can do with the super resolution data, is we can load the cell up in time. So you can see these fluorescent dyes that have come on in time. So I think Alex and Alex, from LUME had both mentioned that there's different dimensions in which you can load the cell up in X, Y, Z time, intensity and that's for people to play around with.
Julia - Nice. And, what specimen would you like to walk in next? You've done the T-cell. What would be next on your list?
Anoushka - I think there's a number of things, bacteria would be great to have a look at neurons, but I think first of all, I'd love to be able to walk around in some brain tissue. I think that would be really powerful, especially for lots of neurological diseases that are affecting a lot of the population. So Alzheimer's, Parkinson's and I think it'd be really exciting to know a little bit more about how we function.
Julia - Yeah, definitely. And especially people who have genetic conditions where that affects proteins on cells and you can maybe tag them and see what's exactly different in the brain and be fully immersed and that, I think that's amazing, but for you, how did it feel to be fully immersed?
Anoushka - To be honest, it was an amazing experience and also really exciting for understanding the future, of our bodies and how we work. Just like Leeuwenhoek did, but in even finer detail.
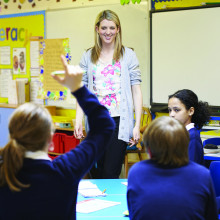
54:53 - QotW: Does the moon affect student behaviour?
QotW: Does the moon affect student behaviour?
There are many fictional tales of werewolves; people who become feral and change their behaviour during a full moon. Bus is there any grounds of science for it? Otis Kingsman reached out to Teresa Pavia', neurologist and the clinical director of the Sleep Medicine Centre in Lisbon to find any connections between the moon and the behaviour of school students...
Teresa - Hello everybody. I am Teresa Piva, a sleep specialist from Portugal. A study in Germany in adolescents from 14 to 17 years of age displayed no lunar periodicity in objective physical activity, subjective sleep quality or time in bed.
Otis - Pretty clear cut answer. Well, Teresa thinks there's a bit more to it.
Teresa - The sweet light of the moon enabled nocturnal light to our ancestors. However, it seems to have important effects for sleep. It enables reducing each duration and its efficiency. Furthermore, indigenous communities in Argentina were compared with college students in the United States. In both groups sleep starts later and is shorter on the nights before the full moon, when moonlight is available following dusk.
Otis - That's the key part of this question, sleep. Students who don't get enough sleep often find the school experience to be more difficult. Tasks that involve planning are most impaired by tiredness. Just how important is for moon in this equation?
Teresa - A multinational study from 9 to 11 years old in 12 countries found that sleep duration differs significantly between moon phases. In fact sleep was five minutes per night, shorter during the full moon compared with the new moon. In fact, the difference is very small and the insignificance in practice is questionable.
Otis - Sleep deprivation plays a key part in a child's behaviour at school. And whether it's caused by the moon or by other outside factors, it's still something we must be aware of. Thank you to Sal for submitting this question.
Comments
Add a comment