This month we’re zooming in on cancer, finding out how researchers are tackling tumours in unprecedented detail. Plus, our gene of the month is the guardian of your genome, rather than the galaxy. This is a shorter version of the main Naked Scientists podcast Zooming in on Cancer.
In this episode
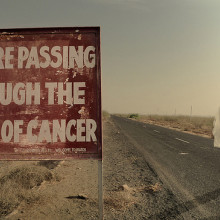
00:47 - What is cancer?
What is cancer?
Dr Emma Smith, Cancer Research UK
Around the world, more than 14 million people are diagnosed with cancer every year, and that figure is expected to rise to more than 21 million by the year 2030 so it’s a growing global problem. Kat Arney spoke to Emma Smith, science communications manager at Cancer Research UK, to get the basics about cancer.
Emma - Normally cells are very highly controlled. They grow only when your body needs them and when there are signals to do so. Now in cancer, some of these signals, some of the genes in the cells have gone haywire. For some reason the cells start dividing and multiplying out of control and then you have too many of a certain kind of cell, and that’s when you get a tumour forming.
Kat - Are we talking about cancer as one disease? There is the ‘big C’ - is it just one kind of thing?
Emma - Absolutely not! We are made up of hundreds of different types of cells and cancer can appear in lots of different tissues: the bowel, the lung, the pancreas, everything and all these different types of cancer have different characteristics. They’re caused by different faulty molecules and different faulty genes and, of course, that will require different treatments. So we absolutely cannot treat cancer as one type of disease.
Kat - What do we know about what causes different types of cancer? People blame things in the environment, people blame stress, some people say well, it’s just in your genes isn’t it?
Emma - It’s a really, really complicated mixture of all of it. Some of it is down to things that we do, ways we live our lives, things in our environment. We call these preventable causes of cancer. These are things in our environment that can cause damage to our DNA, and it’s this damage to our DNA that introduces a fault and the faulty gene can accidently tell the cell to start multiplying and, bingo, you’ve got a cancer.
But, of course, that’s not the end of the story. Some of cancer is down to - I’ve seen it called in headlines “bad luck.” Well, let's not call it bad luck, let's just call in nature instead. All of our cells are capable of dividing and, every time they divide, unfortunately, as much as we would like to believe it, humans aren’t perfect. As a cell copies itself and copies its DNA, it can just accidentally introduce a mistake. It’s not a 100% perfect process and just by doing so, again you can end up with a fault in a key gene and possibly cancer developing. So that’s the kind of nature side of it.
But chemicals and things in our environment can also introduce these DNA mistakes, so it is a mixture of both. Around 4 in 10 cancers are, let's call them “preventable,” so there are lots and lots of things that people can do if they want to reduce their cancer risk.
Kat - You’ve mentioned faulty genes and molecules can drive cancer. So how much cancer is actually in the genes? Can it be inherited - what’s the story there?
Emma - It can be inherited. There are certain examples where people inherit a fault from either of their parents and this particular gene is a really important and, therefore, it increases a risk of cancer. But this is really a minority of cancers. Most cancers are simply a result of nature, or simply getting older. Because damage builds up in our cells over time; all those things that I mentioned in our environment. So there are certains genes, but these form a very small proportion of cancers that we see.
Kat - So, so far we know that cancer is not one disease; it’s many different diseases. There are complex causes. There’s an interplay between the things in our environment, the things we do, and the things in our body itself. But then how do we treat cancer? Presumably, it’s not one size fits all for treatment either?
Emma - Absolutely not, but treatments can be broadly grouped into categories. Drugs that often make the headlines and modern drugs are very expensive. But these drugs aim to specifically home in and target cancer cells; they’re called targeted therapies. Chemotherapy, which is one of the cornerstones of cancer treatment. It’s more general in that it’s not specifically designed just to hit cancer cells but it is, in some cases, very, very effective. The other is radiation treatment, so radiotherapy and, of course, surgery. Put them all together and, usually, patients receive a mixture as well. They don’t just receive one therapy; normally it’s a combination of several different types of therapy.
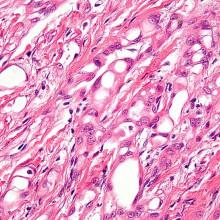
06:09 - Building tumours in the lab
Building tumours in the lab
Professor Fran Balkwill, Queen Mary University of London
Cancer causes tumours, but tumours aren't just made out of cancer cells, as Kat Arney found out when she went to visit Fran Balkwill, Professor of Cancer Biology at Barts Cancer Institute, Queen Mary University of London, who is working on ovarian cancer, which spreads inside the body, particularly into the peritoneum - that’s the lining inside the abdomen - and the omentum, the scientific word for tummy fat.
Fran - My interest is the entire cancer. A lot of people think that cancers are born of malignant cells that have gone out of control. And this is true but that’s only half the story. When we think of really, probably all cancers, then they are actually a mix of the malignant cells, the cancerous cells, but a whole host of other cells that the cancer has recruited and, in some ways, corrupted to help the cancer grow and spread. 50% or more of any lump that is a cancer can be these normal cells that are promoting the cancer growth and spread.
Kat - What type of cancer are you looking at?
Fran - We’re looking at a type of ovarian cancer called high grade serous ovarian cancer. What you find when you look is that you find a mixture of fat cells, which are in parts of the peritoneum, and we think the cancer can use that fat to help them grow. Then you find fibroblast builder cells, you find new blood vessels, you find lot’s and lot's of immune cells that really should, and we know they could, fight the tumour but they don’t, and the malignant cells as well. There’s up to 20 or more different cell types actually in a cancer alongside the cancerous cells.
Kat - But certainly from my experience of working in a lab, you just look at cancer cells growing in a plastic dish but, obviously, that doesn’t capture all this diversity you’re talking about?
Fran - No. Absolutely and that’s the problem. Now that’s why we use mouse models of cancer because then you can in a living organism, but sometimes we need to have all human cells. Some of the new treatments actually have antibodies that act against human cells and not mouse and so we really need to compliment the mouse models, we need to have some human models. So how do we set about this?
Kat - The human lab…
Fran - Yeah. The human lab. We are not made of plastic and cancers are more than just cancer cells growing on a plastic plate. But with all the advances in bioengineering and regenerative medicine we think, and many people are trying to do this now, that we can begin to build complex three dimensional models of more than one cell type. More than just a cancer cell and answer some of these basic questions about how tumours form as well as what new treatments we can use.
Kat - How do you go about doing that then? How do you about reconstructing all this three dimensional glory of a tumour in the lab?
Fran - It’s with great difficulty I think I would say. But the approach that we’ve taken is to first deconstruct, so we get from surgery the omentum, which is part of the peritoneum from patients with high grade serous ovarian cancer.
First we found out many things, not all of them, but many things that we thought would be useful so that we would have a kind of template for a model, but also we would be able to validate. We would be able to say how close is any model we build to the real thing. So we did that for quite a long while and that’s proved extremely useful and also rather interesting. We learnt things about the tumours that we didn’t know before when we were just looking at the cancer cells.
Now we’ve taken various approaches; the most promising is to build an artificial omentum because in normal people the omentum is mainly fat cells. So we’ve been able to grow an artificial omentum and we have some idea how stiff it should be from the deconstruction and we have some idea about what other cells are there. Then we are growing the tumour cells, and the fibroblast builder cells, and also some other cells called mesothelial cells and we’re trying to recreate a model where the tumour is first invading that omentum. And also another model where the tumour is already there in situ with all the cell types.
We haven’t got all the cell types yet and the next challenge is the immune cells. But we’ve got some of the major cell types and all from the patient putting together and now we’re just testing to see how close those cell types resemble, in some of their functions and things that they make and things they do, the tumours that we take from the patients.
Kat - So it’s a bit like taking a house and unbuilding it brick by brick, taking everything apart, the beams. the bricks, the insulation, looking at what you’ve got and then trying to reconstruct that again?
Fran - Yeah. I think that’s not a bad analogy. It’s very complicated. We’re never going to be able to completely recreate the cancer obviously. The important thing now is to find questions that we cannot answer in any other way, or we need to complement our studies in the mouse models and then work out what combination of cells we think can answer those questions.
Kat - So now you’re managing to grow these little tumours, these little systems in the lab, what’s the ultimate goal? What are you going to do with them?
Fran - First we need to check that they do enough to resemble the human tumour and then, from our point of view, we want to find new treatments that disrupt the interaction between the malignant cells and all those cells that they corrupt and recruit because, if we can do that, we can break of some of the supply lines of the tumour. We also want to use them to understand how the tumour cells and the other cells interact to form tumours in the first place. So it’s understanding some basic cancer biology, but also a complementary way to the other ways we have of testing new treatments.
Kat - I look at some of the stories that are coming out of world of tissue engineering, and researchers using things like 3D printers to think about making new organs and all this kind of stuff. What would be your dream for making these kind of tumours in the lab?
Fran - Well, I think that you’re absolutely right, and we’ve just got some funding to get a 3D bioprinter. It’s going to take us a while; it’s not going to happen overnight but I think it’s a nice idea. I was talking about this to a load of clinicians the other day, oncologists. One came up to me afterwards and said “do you know when you were talking about that, I had a vision of a bioprinter printing little tiny living people.”
Kat - That would be the ultimate model, wouldn’t it?
Fran - Yes. Unfortunately there’s something about the size of cells that make us the size we are and, unfortunately, you can’t make tiny people but it’s just a wonderful idea.
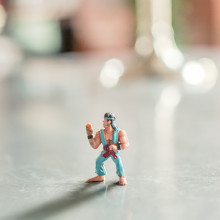
14:20 - Gene of the Month - p53
Gene of the Month - p53
It’s time for our gene of the month - p53, also known as the Guardian of the Genome.
The story starts back in the 1970s when a British scientist called David Lane was working with his colleagues at the Imperial Cancer Research Fund (now Cancer Research UK) studying a monkey virus, known as SV40, which caused cancer in mouse cells grown in the lab.
In particular they were looking at two proteins made by the virus – known as the “large T” and “small t” antigens, believed to be the key to its cancer-causing abilities. By trawling infected cell extracts with these proteins, in the same way a fisherman might trawl the sea with a net, Lane hooked out a small protein weighing just 53 kilodaltons (that’s the unit of measurement for molecules).
Writing in their groundbreaking 1979 paper, published in the journal Nature, Lane and his boss Lionel Crawford note that “It is possible that [the mystery protein] … may normally act as a regulator of certain cellular functions related to growth control…It is of prime importance to determine the level of this … protein in [normal] cells and to see if it is induced by other carcinogenic agents.”
The human gene that encodes p53, called TP53, was found in 1984 and we now know it’s responsible for turning other genes on in response to DNA damage. You can see your own TP53 gene at work if you’ve ever been foolish enough to get sunburnt – that horrible peeling stuff is dead skin cells, killed as a result of p53 switching on a cell ‘suicide’ pathway preventing the damaged cells from turning cancerous - hence its nickname, “the guardian of the genome”. That’s great when it’s working, but the majority of cancers seem to switch off the gene or somehow inactivate the protein, effectively throwing the guardian in jail and running riot in the body.
Since its discovery, p53 is now known to be involved in a huge range of biological functions, from ageing and immunity to development in the womb. Nearly four decades on from David Lane’s first discovery, scientists are still trying to figure out exactly how it works inside cells, and how to use that knowledge to tackle cancer and other diseases.
One approach is to use drugs that can reactivate p53 in cancer cells - letting it out of prison so it can kill the rogue cells. Another approach is to specifically target and kill cells that don’t have active p53 - which should be cancer cells, rather than healthy ones. So far this is all at an experimental stage, so watch this space.
And speaking of Guardians - but of the galaxy rather than the genome - as far as I can tell, there are currently no genes named Groot or Baby Groot, so they’re still up for grabs in case you’re a geneticist in search of ideas for your latest DNA discovery.

31:48 - Zooming in on micro-RNA
Zooming in on micro-RNA
Peter Leedman, The University of Western Australia
As well as studying the genes involved in driving cancer and using this information to drive the development of new cancer drugs that target faulty proteins, researchers are also taking a more direct approach, investigating how to turn down the activity of genes themselves. One of them is Professor Peter Leedman from the Harry Perkins Institute of Medical Research at the University of Western Australia in Perth, who explained his ideas to Chris Smith...
Peter - We’re fascinated with the concept of using part of the genome. That is the DNA blueprint as a new therapy in cancer. And, in particular, some of the solid tumours like liver cancer, pancreatic cancer, head and neck cancer, and brain cancer, for which there are very few treatments, and the prognosis and the outlook may be very poor.
Chris - We know cancer is a genetic disease. It’s when genes go wrong and, therefore, cells begin to obey the wrong sets of instructions or disobey the normal sets of instructions. So when you’re say you’re trying to use the genome to come up with a new way to treat cancer, what do you mean?
Peter - Some of these solid tumours, they are driven by factors such as receptors that sit on the outside of the cell, and they drive the cells to proliferate or grow in an unrestrained way. We’re looking at that as the set of targets. The second part of the story is saying what can we discover inside the genome or the DNA blueprint that might give us an answer for slowing down that receptor which drives the growth. And the dogma for 50 years was that a lot of the DNA was said to contain junk, so called “junk DNA.” What’s been discovered over the last 15 years is that about 2% of the DNA is really important and made into protein, but that 98% of the non protein-making DNA is really important.
Chris - Just to clarify that a bit then. So you're saying that 2% of the DNA code actually are genes, and those genes turn into proteins in cells; they’re like recipes that make proteins. The 98% is really important but how? What is the 98% making then, or doing that makes it so important?
Peter - The 98% tends to make what we call non-coding RNA. So DNA is made into RNA - this copy - and it’s that copy that’s not turned into protein in the 98% of the genome, and it’s that that contains this rich tapestry of little molecules called RNA. And that is an area that’s of great interest globally because we believe that if we can untap some of the secrets in that 98% of the DNA, so called junk, that some of them might be useful for clinical treatments.
Chris - Right, so the dogma of for what’s useful about DNA is that it contains recipes that make proteins. That’s going out the window and what we now realise is that it might not be making proteins but this DNA sure as hell is making something important. It’s making these RNA molecules and what, do they stay in the cell and do something?
Peter - What we know: some of them are really small, only 22 or 23 bases long. What we found is that one of them called microRNA-7 is particularly powerful at slowing down and inhibiting the growth receptor that drives a number of these cancers, and it does it in a very sophisticated way. It basically takes out the key receptor itself and multiple friends of the receptor that help drive the growth and promote the tumour, and what we find when we add microRNA-7 to a number of these cancers, we can stop the growth of the cancer in the test tube. We can also stop it in mice and that’s particularly exciting.
Chris - What do they do physically inside the cell to achieve that effect? Do we understand chemically what they are doing?
Peter - What we know is that the microRNAs work inside the cell and they target the message and basically blow it apart. That protein being a key growth receptor that drives the cancer, that now is taken out of the cell and so the cancer undergoes what we call cell death.
Chris - So it’s a way of specifically, and in a highly targeted way, taking down a discrete genetic message so it robs the cell of the ability to have that genetic message that it was making too much of to make it at all? The only problem is that that would happen in every cell in your body, if you just deluged a person in this microRNA-7, they would be robbed of the ability to make that gene product everywhere. And that would be bad, wouldn’t it?
Peter - That’s a great question. What we know about these tumours, these nasty tumours such as liver cancer and head and neck cancer, for example, is they’re characterised by having lots, and I mean sometimes millions, of these growth promoting receptors on the surface of the cell. In contrast, normal cells which might have a thousand or much, much lower amounts and the principle here is to replace back something that the cancer cell used to make, and that’s a really important point.
The cancer cells we’re looking at used to make microRNA-7 and they’ve lost it and it provides this incredible break on growth promotion, and when you lose it you provide the opportunity for the cell to grow in an unrestrained way. Now, if we add back microRNA-7 it targets the growth receptor promoting pathway and all it’s friends in that, but it typically will target those cells in the body that have lots, and lots of the receptor. Much, much more than the normal cells, and so we believe that’s why it would be better tolerated and, of course, fight the cancer very effectively.
Chris - And you can get this message into the cell sufficiently, can you?
Peter - We can. There is, when we give the microRNA intravenously in model systems, it’s very well taken up into the cancer. So we believe this will be a wonderful way of treating human cancer.
Chris - One of the things that cancer is notorious for is it’s genetic diversity. And one of the reasons why cancers respond poorly in the end to chemotherapy is because, eventually, you get cancer cells that have evolved and been selected out, and they have an ability to rid the cell of whatever the agent is you're putting into it to try and kill it. So, is there a possibility that all you’re doing is kicking the can down the road and we’re going to select using your agent cancer cells that can just make even more of the gene for the receptor and you'll be back where you started?
Peter - Great question. One of the key things in cancers is they often develop resistance to the first therapy or therapies that they might be given. One of the things we’ve found with microRNA-7 is that when we give it to cells that have become resistant to a chemotherapy agent such as cisplatin, which is often used for head and neck cancer, we find that we can re-sensitize the cells to the chemotherapy agent by giving microRNA-7.
That’s really exciting because that suggests that we can rescue patients from the terrible traumas of resistance. And in real terms, when cancer kills people, it kills people because it comes back and it comes back in dreadful areas and spreads all over the body. You don’t die from the first time you get a cancer, you’d actually die from the complications of the cancer occurring - so called metastasis or metastatic disease. And we’re very excited about this molecule, microRNA-7, because of it’s ability to work with other agents and make the tumours resensitized to those agents and therefore, hopefully, improve the outcome.
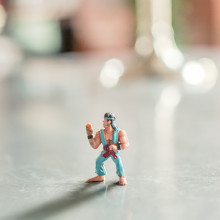
39:38 - Gene of the Month - p53
Gene of the Month - p53
It’s time for our gene of the month - p53, also known as the Guardian of the Genome.
The story starts back in the 1970s when a British scientist called David Lane was working with his colleagues at the Imperial Cancer Research Fund (now Cancer Research UK) studying a monkey virus, known as SV40, which caused cancer in mouse cells grown in the lab.
In particular they were looking at two proteins made by the virus – known as the “large T” and “small t” antigens, believed to be the key to its cancer-causing abilities. By trawling infected cell extracts with these proteins, in the same way a fisherman might trawl the sea with a net, Lane hooked out a small protein weighing just 53 kilodaltons (that’s the unit of measurement for molecules).
Writing in their groundbreaking 1979 paper, published in the journal Nature, Lane and his boss Lionel Crawford note that “It is possible that [the mystery protein] … may normally act as a regulator of certain cellular functions related to growth control…It is of prime importance to determine the level of this … protein in [normal] cells and to see if it is induced by other carcinogenic agents.”
The human gene that encodes p53, called TP53, was found in 1984 and we now know it’s responsible for turning other genes on in response to DNA damage. You can see your own TP53 gene at work if you’ve ever been foolish enough to get sunburnt – that horrible peeling stuff is dead skin cells, killed as a result of p53 switching on a cell ‘suicide’ pathway preventing the damaged cells from turning cancerous - hence its nickname, “the guardian of the genome”. That’s great when it’s working, but the majority of cancers seem to switch off the gene or somehow inactivate the protein, effectively throwing the guardian in jail and running riot in the body.
Since its discovery, p53 is now known to be involved in a huge range of biological functions, from ageing and immunity to development in the womb. Nearly four decades on from David Lane’s first discovery, scientists are still trying to figure out exactly how it works inside cells, and how to use that knowledge to tackle cancer and other diseases.
One approach is to use drugs that can reactivate p53 in cancer cells - letting it out of prison so it can kill the rogue cells. Another approach is to specifically target and kill cells that don’t have active p53 - which should be cancer cells, rather than healthy ones. So far this is all at an experimental stage, so watch this space.
And speaking of Guardians - but of the galaxy rather than the genome - as far as I can tell, there are currently no genes named Groot or Baby Groot, so they’re still up for grabs in case you’re a geneticist in search of ideas for your latest DNA discovery.
Comments
Add a comment