There's more to life than the four letters of DNA, and our cells use a chemical tag known as DNA methylation to mark out certain parts of the genome, helping cells to remember what they're doing. And, as you might expect, it's pretty important. Plus, how your GCSE success could be encoded in your genes, an important molecular cause of autism identified, and an illuminating gene of the month.
In this episode
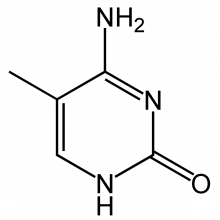
01:07 - Peter Jones - DNA methylation
Peter Jones - DNA methylation
with Peter Jones, Van Andel Institute, Michigan
Kat - This month I'm reporting back from a fascinating meeting I went to up in Edinburgh, a Wellcome trust Waddington Symposium entitled "Epigenetics in dialogue with the genome". We tackled the wonderful world of epigenetics in last month's podcast, exploring some of the ways in which genes get turned on and off during development and disease, and now it's time to turn our attention to one of these marks in particular: DNA methylation.
As has become increasingly clear over the past few decades, there's more to life than the four chemicals letters, or bases - known as A, C, T and G - that make up DNA. These letters can be modified in certain ways, primarily by the addition of a small chemical tag known as a methyl group to the letter C. This modification is known as DNA methylation, and it's thought to play an important role in controlling how our genome gets used during normal development, life and disease.
To get the low-down on the mysteries of methylation I spoke to one of the leading experts in the field - Professor Peter Jones from the Van Andel Institute in Michigan.
Peter - The presence of 5-methylcytosine, in other words, covalently modified extra base in DNA, was really found I think more than 60 years ago. But exactly 40 years ago in 1975, there were two papers that appeared - one by Robin Holliday who was here in England and one by Arthur Riggs who was in Southern California, postulating that these chemical marks on DNA could have information coding properties. In other words, they could specify which genes would be used and which ones would not be used. And very importantly, in these prophetic papers, they proposed a mechanism for inheritance. So, the idea was that as you know, you inherit your genes and then methylation patterns put on the DNA during development which tells cells what they should do and what not to do. Just like the genes could be replicated, the methylation pattern can also be copied and replicated. So, these two papers really stimulated the field. I got involved in the field completely by chance. I was working with a drug which was developed in Prague, in Czechoslovakia in the late '70s. We found that this drug could change the differentiated state of cells very markedly.
Kat - So, it would make them forget what they were meant to be doing.
Peter - Well actually, better than that. You know, telling them to do something else. They didn't only forget what they were but they did something else. Actually, they were non-descript cells growing in a culture dish and we could change them into beating muscle and fat and cartilage. So, we'd had no idea how this could possibly be. It was very unusual. But then when we looked into it in more detail, we discovered these papers that I just told you about and we're able to figure out that the way these drugs worked is they worked as erasers. They got into the DNA, they removed the methylation from the DNA because they inhibited that process, and then the cells went ahead and did their thing, and put in new methylation patterns and became either muscle, fat or cartilage. And so, these experiments and those of Adrian Bird and others were really important in showing two things. First of all, that the patterns could be inherited and secondly, that they could be changed. And if you changed them, it had major impact on the cells.
Kat - One of the ways I really like thinking about DNA methylation, it's almost like a kind of post-it notes in the recipe book that kind of cells remember somehow that they're going to use these genes and not use that gene, and they need to keep remembering to do that. Where have we come from those early discoveries that there were these kind of marks on DNA and they could be taken off, and that cells could then do new things?
Peter - Well, those were really hypotheses and they turned out to be essentially correct. So, I think what we've done in the last few years was figure out how the patterns are copied. We've worked out how these patterns are really important in telling cells apart from each other. We have found much to our surprise that these patterns are fundamentally altered in human cancer and in fact can be used to silence genes which are important in the development of cancer. So that in fact, cancer can develop because its methylation patterns are altered. And so, it's been a really interesting few years. Also, more recently, the drug that I just told you about - 5 azacytidine - is now being used in patients to treat cancer. And so, we've gone the full loop from an idea in 1975 to 30, 40 years later actually having drugs in the clinic which can be used to treat cancer in people. So, it's a very exciting time.
Kat - What do you think are still some of the - I guess, the known unknowns, the questions that you still really want to answer?
Peter - I think the questions are still very, very substantial. We don't know the very, very fine detail of exactly how cells can put these patterns on in a precise manner. We don't know exactly how the cells can recognise the patterns. We know they're altered, we know they're there, we know they're useful, we know they're deranged in cancer, but we don't know all the real details of exactly how these patterns are applied.
Kat - With DNA methylation, it's a mark that's put onto DNA. It could be copied as cells divide. What do we know about the possibility that it could be even passed on say, from parent to child because this is a really exciting but quite weird area, I guess?
Peter - Yes, it is exciting and I would also say it's not at all clear the extent to which that really happens. I think that there is a lot of interest in that area, particularly with the public. The public likes to hear about things like that, you know. But I think the evidence at the moment is in my opinion, unconvincing. We have a long way to go to be sure whether in fact that can happen because what happens is, when cells get through the germ line, when they make the sperm or eggs, the methylation patterns are essentially erased. They reset, they rejigged in such a way that you can make a human being. As part of that process, most of the changes that might have occurred earlier on are actually erased. But there are people that believe that this can happen and I would say the jury is still out.
Kat - The other things that we sometimes see in the paper, we hear the word epigenetics, we hear about maybe DNA methylation and it might be possible to change it, for example, influence it through what you eat or drink. What's the evidence on that? Can I pimp my genome through my diet?
Peter - That's a very good question as to where the diet influences the epigenome. So yes, there are experiments which seem to show that. There have been experiments done in pregnant mice for example which show that if you alter the diet of the mother, you could change methylation patterns and influence various properties of the mice. But the generality remains very, very unclear. And so, there's a long way to go, again, very controversial area. Some people believe in it, others don't.
Kat - So I guess, if a good scientist, we have to do the experiments and get the data.
Peter - Yes, I would absolutely agree that that's necessary.
Kat - How do you feel about working in this field that's taking our understanding on how genes works so much further than the four letters of DNA into this sometimes seemingly, almost magical world of taking the genome that's in all our cells and then using it in so many different ways?
Peter - Well, I found it extremely exciting, the fact that we can now begin to understand to a certain extent how this works. But yes, it's very, very exciting and then to understand its ramifications, not only in normal human development but also in abnormal development and in late onset diseases which is what I think we now need to look at. Is there a role for changing DNA methylation patterns in the genesis of those diseases? We don't know, but we're beginning to look.
Kat - That was the Van Andel Institute's Peter Jones, and we'll be hearing from one of the scientists he mentioned - Adrian Bird - later on.

09:52 - Robert Plomin - GCSE genes
Robert Plomin - GCSE genes
with Robert Plomin, Kings College London
Kat - We often talk about things being "in the genes", from traits such as eye or hair colour to our risk of diseases. One of the main ways that scientists figure out how much a particular characteristic is down to genetics - known as its heritability - is by comparing identical twins, who share 100 per cent of their genes, with non-identical or fraternal twins, who only have 50 per cent of their DNA in common. Thanks to a unique study tracking thousands of pairs of twins as they grow up, Professor Robert Plomin and his team at King's College London have now discovered that genetics makes an unexpectedly large contribution to children's GCSE grades across a wide range of subjects.
Robert - In this twin study which we call the Twin's Early Development Study which is a study of about 7,000 pairs of twins in the UK, I was interested in focusing on an area that hasn't been studied much and that's school achievement. So on the one hand, we know that cognitive ability like intelligence shows substantial genetic influence. But people hadn't really studied the business end of it in terms of school achievement. And so, we were surprised to find from the very first years of school that school achievement as measured by the national curriculum scores. It's very highly heritable, like 60 per cent heritable. That means, of the differences in children's performance in the national curriculum test, over half of those differences between children are due to DNA, genetic differences, between them. So, we're not identifying the DNA, but we're using the twin method to estimate, not only the significance, but the effect size of genetic influence on school children. It's very high.
So, we've been following them all along and now that they hit 16, we wanted to use the GCSE scores - there aren't many countries where the same national tests are administered to everybody. And so, what we've found is the same sort of thing that GCSE scores are highly heritable. But what's new is that all the tests - there's over 80 subjects that people can take for GCSEs - and all of them are highly heritable. That surprised me because I would've thought the STEM subjects - science, technology, engineering, math - would be more heritable for some reason maybe because it involves intelligence to a greater extent than drama and art. This is just totally exposing my biases of course as a scientist, but it wasn't true. They are all equally heritable. It's interesting that scores are as equally heritable despite the fact that some children are getting tutors and going to schools that have prepped them for GCSEs. Schools - we make a big deal about schools - you just say what school our kids in explain far less than 20 per cent of the variance. Explaining 50 per cent of the variance with genetic differences is extraordinary when education totally ignores genetics. In teacher training or whatever, not a word is said about genetics. And so, I'm just saying genetics is very important.
But what's really novel about this study is a little bit harder to understand and that is to say, "Okay, genetics affects all of these GCSE subjects" but is it different genes for every one? Are there genes for drama, genes for music, and genes for math? And the answer is definitely not. The same genes are affecting performance on all of these GCSE scores. The differences are probably more environmental. If you're good at drama and not good at math, that's probably more of an environmental thing. But the genetic action has to do with what's in common in performance across all of these things.
Kat - Is it not just that they're just generally smart? They've got good intelligence genes?
Robert - That's what most people would say and so, what we did is we took out intelligence. We corrected for intelligence. You can correct scores for age and sex, and you can correct scores for intelligence. So, you can take these GCSE scores and make them independent of intelligence, statistically. And then the interesting thing was that we got the same results. So, everything is equally heritable, independent of intelligence, and what's even more surprising, again, it's the same genes that affect all of those intelligence corrected GCSE scores. So what that means is that, your hypothesis is a good one that a lot of what the genetic correlation among all these GCSE scores is about intelligence. But what's amazing is you take out intelligence and you find, yes, there's still genetic influence, but it also works in a very general way and that's suggests it's like an academic ability, genetically driven academic ability.
Kat - When you say that this ability, this academic ability is heritable, does this mean that we can pin it down and say, "It's this gene. It's that gene. It's this gene"? Can we find these genes?
Robert - Well, a first step in trying to find genes is to find something that's heritable. The research over the last few years is saying that cognitive abilities and now school achievement is highly heritable, motivates people to try and find genes. But what we know so far from all of the life sciences is that for complex traits - that it's not for the thousands of single-gene disorders that are very rare, but for the common disorders in medicine like cardiovascular disease or obesity, or psychiatric things like alcoholism and depression - they're heritable but they're not due to one gene by any means. We're thinking now there's thousands of genes of very small effect which means it's going to be extremely difficult to identify the specific differences responsible for the heritability. But it's all part of a package. It would be nice if we're just talking about a handful of genes - the genes for math or something like that. But if this is what we're finding, then we're just going to have to roll up our sleeves, get the strategies that will allow us to identify, not the gene or the few genes, but thousands of genes that are responsible for the heritability of these complex traits.
Kat - King's College London's Robert Plomin, whose study came out in the journal Nature Scientific Reports. We'll be looking in more depth at his twin study - as well as other large-scale genetic studies - in next month's podcast.
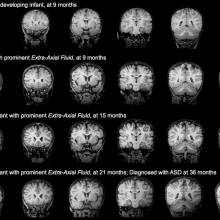
15:54 - Mark Zylka - Autism gene advance
Mark Zylka - Autism gene advance
with Mark Zylka, University of North Carolina
Kat - Recent advances in genetics have allowed researchers to start pinning down thousands of gene faults or mutations involved in a wide range of diseases and disorders, from cancer and heart disease to schizophrenia or depression. A gene recently linked to autism encodes a protein called UBE3A, which appears to control how nerve cells in the brain connect to each other. The levels of UBE3A are normally controlled by a kind of molecular switch known as phosphorylation - which involves attaching a chemical tag to a site on UBE3A, switching it off. But what happens when that switch can't be thrown and UBE3A can't be turned off? The result is autism, according to University of North Carolina researcher Mark Zylka.
Mark - Basically, as with many things in science, we got lucky. There's really a revolution that's taking place in the area of autism genetics and this is a revolution that's really just begun a few years ago. There are several large groups, large consortia, that are sequencing the genomes of individuals with autism and their unaffected parents, and they're searching for genetic mistakes in the kids that are not present in the parents. What they're finding are a large number of these new mutations that are present in the children with autism that are not present in the parents.
Two of these studies were just published in December. In these studies, there are literally thousands and thousands of mutations. One of them was in UBE3A. I sent that information to Jason Yee, a post-doc in my lab and I asked him, "Could you tell me where is this mutation in UBE3A?" A couple of hours later, he basically came back to me. He had this huge grin on his face. He said, "You're not going to believe this, but that mutation is in the phosphorylation site that prevents phosphorylation." And so, that was really the 'eureka' or the 'aha' moment, but as with anything, we wanted to really confirm this.
This material is available so we were able to obtain the cells from the child and the parents, grew them up in our lab and then sequence to confirm that that child did indeed have that mutation whereas the parents did not and then we were able to get UBE3A extracted from those human cells and show that the individual with autism had a hyperactive version of UBE3A whereas the parents did not. And so, that was really unprecedented for us to be able to do something like that.
Kat - We know that autism is a neurodevelopmental disorder. It causes changes, problems in the brain. Were there any signs that having this overactive protein might be affecting any of the cells in the brain?
Mark - We believe, yes. And so, the way we looked at that was to take this hyperactive version of UBE3A and insert it into the brain of an animal model in a subset of cells, a subset of neurons, and then we looked at those neurons that contain this hyperactive version of UBE3A and looked at the number of spines or dendrites on the neurons. Spines make up synapses and synapses are essentially the points of communication from one neuron to the next. What we noticed was that the neurons that took up this hyperactive version of UBE3A had many more spines than the neurons that did not. This again, really points a finger towards autism because of autopsy studies that have been done - one of the hallmark features of individuals with autism is an excess of spines in their brain.
Kat - Potentially, what proportion of cases of autism are we talking about being caused or being involved with this kind of genetic fault?
Mark - It's about 0.25 per cent of all patients with autism. That may seem like a small number, but this actually represents the third most common chromosomal abnormality in patients with autism. So, it's a fairly common form of autism.
Kat - I'm sure that people who have children with autism will be thinking, okay, so where are some cures then, where are some treatments? How could this work pave the way for maybe treatments that could help children with autism?
Mark - What this work suggests is that targeting UBE3A, turning off UBE3A might represent a new way to treat autism so we can take advantage of this phosphorylation event that could in turn tamp down the activity of UBE3A and hence, provide a therapeutic benefit.
Kat - It sounds like genetics has really changed our understanding of autism and the way that we research it. How do you see this revolution continuing?
Mark - Just like with cancer, there are many different types of cancer. There clearly are many different genetic types of autism. Sequencing individuals with autism is going to be the future. So, we're no longer lumping them altogether as individuals with autism but as individuals with certain forms of autism.
Kat - Mark Zylka from the University of North Carolina, and that research was published this week in the journal Cell.
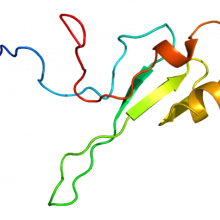
21:19 - Adrian Bird - Hope for Rett Syndrome
Adrian Bird - Hope for Rett Syndrome
with Adrian Bird, University of Edinburgh
Kat - You're listening to the Naked Genetics podcast with me Dr Kat Arney. Still to come, we'll be lighting up our lives with the gene of the month. But first, it's time to take another look at this month's topic of DNA methylation, or more specifically, Rett Syndrome - the rare but severe genetic disease caused by defects in the gene encoding a protein called MeCP2, which sticks to methylated DNA. Professor Adrian Bird, from the University of Edinburgh, has dedicated his career to studying DNA methylation and the molecules that recognise it - and is hopeful that his research might one day lead to new treatments, or even a cure for children affected by Rett syndrome.
Adrian - Rett syndrome is an autism spectrum disorder which is very distinctive. It was discovered by Andreas Rett in Vienna. But it is caused by mutations in a gene. So, it's a genetic disorder, but it just so happens that the gene that is mutated encodes a protein that is involved in epigenetic reading of the genome and specifically, what that protein is supposed to do is bind to methylated sites on DNA which is a methyl group. It's just like a little nutty knob that you can stick onto DNA and MECP2 recognises and binds to that. In the absence of that protein, the brain does not work properly.
Kat - So this protein, MECP2 is kind of recognising this mark on the DNA, involved somehow in turning genes off inside cells. What do we know about how this genetic fault is affecting brain cells and how are you trying to understand this disease and bring forward some treatments perhaps?
Adrian - Well, that's what makes - obviously, there's the human issue of dealing with the consequence of this that one would like to fix. But the attractive thing from a research perspective is that here, you have a complex disorder that affects cognition, understanding, behaviour, etc. and you know exactly at the molecular level what the cause is. Now, what one is trying to do is take little steps that you understand in going from the mutations that affect this protein to those complex outcomes and we have not yet joined them up. People are working down from the brain and what's happening in the brain. The nerves do not seem to mature properly. There are groups of people who are trying to work out why not. And then there's the bottom up approach where you start with the gene and find out what the protein interacts with and that's the approach we've largely taken. In the end, the idea is that those coming downwards and those coming upwards will meet. So far, that hasn't happened, but to me, it has all the ingredients that it's a good place to start looking for autism in general and intellectual disabilities, what their genetic roots might be.
Kat - Tell me about some of the results you've had trying to fix or repair this genetic fault?
Adrian - A feature of Rett syndrome is that it's not neurodegenerative. So in other words, the nerve cells, although they're rather simplified, they don't have as many branches as these cells usually do, they are alive. And so, this obviously raises the possibility that if you were to put back the protein, would they become mature now later in the day. The reason why this is an interesting question is because it was widely assumed that it would be too late. I think there's a general view that neurological disorders of all kinds are more or less irrevocable and that's really reinforced by the fact that there are hardly any effective treatments for any of these kinds of disorders. And so, there is the idea that the brain goes through this complex development, it does certain things at certain times and you need all the proteins to be there, and if you miss that, it's too late. You can't go back and redo it. So, we devised a way of testing this idea which was to make an animal model of Rett syndrome that did not have the MECP2 protein and it's a very good model in a lot of respects of the human disorder. And then we put it back. We did this through a genetic trick. So, we waited until the animals were ill and then we switched on the gene. The prediction of the critical period, critical window hypothesis would be that it wouldn't make any difference, but actually, it turned out that they got almost completely better. So, what that means is that there wasn't a critical window where you needed this protein. Actually, not having it makes the brain not work properly and if you put it back, the brain starts to work properly which was a totally surprising result to many people including us, I have to say. What it of course does mean that it raises the prospect that the human disorder will be curable. And certainly, it's fuelled a lot of research in many labs to try to act on that hopeful possibility.
Kat - There must be a huge number of families affected by this disorder who are really desperate for cures. How soon do you think this may get into human studies and are the families really interested in this kind of research?
Adrian - The families are extremely interested in this kind of research. At the moment, there is not meaningful therapy and this offers hope. As for being able to tell them when that hope will be realised, that's of course a very uncertain thing because until you actually have a therapy, you don't know. I could say, it would be very disappointing if in 10 years' time, there was not some therapeutic approach and I would be disappointed. But do I know that in 10 years' time there will be an approach? I don't. There's a lot of work going on and there are some quite hopeful preliminary results, but nothing, I would say, that makes you think, in two years, that will be in the clinic. Of course, getting from an idea or even a drug or a process into the clinic is a pretty difficult thing to do in and of itself even if you have the therapy there. I think all the preliminary work in Rett syndrome suggests that this disorder is one of the most promising ones for there to be therapy in the fullness of time. It's not the only one. Fragile X syndrome is also a common autism spectrum disorder. So, there is hope now as there never has been before I think.
Kat - That was Adrian Bird from the University of Edinburgh.
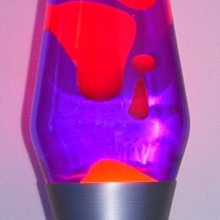
28:17 - Gene of the Month - Lava lamp
Gene of the Month - Lava lamp
with Kat Arney
Kat - And finally, here's our Gene of the Month, and this time it's Lava lamp. Named after the popular and somewhat hypnotic 1960s decorative lighting fixture, Lava lamp was first identified in fruit flies in the year 2000 when researchers were looking for genes involved in helping cells to split in two as they divide in the early embryo. Like the coloured bubbles in the novelty lamp, the Lava lamp protein is located in blobby pockets, known as vesicles, in a special part of the cell called the Golgi body.
Lava lamp seems to interact with another fly gene called Lrrk - a molecular cousin of a gene involved in human Parkinson's disease - and might be implicated in the development of the disease, as Golgi bodies are important for proper nerve structure and function.
Comments
Add a comment