All living cells are made up of protein molecules - but how do they organise themselves into structures? Plus bee sex, tough mice, and a happily married gene of the month.
In this episode
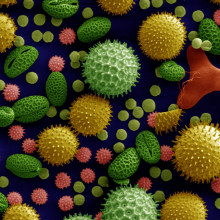
00:58 - Prof Buzz Baum - Genes to shape
Prof Buzz Baum - Genes to shape
with Prof Buzz Baum, UCL
This month I'm reporting back from the Genetics Society Autumn Meeting, which took place at the Royal Society in London. Called "Genes to Shape", the talks brought together researchers ranging from mathematicians and physicists to developmental biologists to discuss how biological shapes are created. To hear more about the idea behind the meeting - and for his summary of the proceedings - I spoke to one of the co-organisers, Professor Buzz Baum from UCL, whose own lab works on cell shape and movement.
Buzz - I think since DNA was discovered and the genome sequence, there's really been a lot emphasis on the gene level and how genes control things. As we know, that's important because if you take identical twins, they look very similar as embryos, as young adults and as people, and old people even. So, if you have identical genes, you really do have an identical phenotype in animals. In plants obviously, they're very different, but genes have a big influence. But I think in a way, the path from gene which is information to form to shape to us, is a very complicated long journey where lots of interesting things happen.
I think what's happened in the community and what this meeting was about is that in a way, trying to understand as you cross the scale from the small bits of information that is in the - these A, T, G, and Cs, this tiny code in the middle of a cell, how that is translated into these amazing large objects, beautifully formed flowers and things which we see in the macroscopic world.
It's very complicated and to understand those processes, you can't just do the conventional kind of science the biologists are taught. You can just take genes and remove them and then you can see what goes wrong. But to understand the process, how you get from small to big growth for example and cell shape changes, you really need to start using tools from the physical sciences which is why we have so many theoretical physicists who are using logical, simple models formulated very simply and then simulations and mathematics to try to see when you put simple elements together, what do you get out.
Kat - What I found was really interesting is, I've been to a lot of genetics meetings where, like you say, people, they look at one gene that's faulty and they find you have a smaller mouse or a bigger dog or something like that. This was going right down to the molecular level, the cellular level. What for you really struck you about some of the talks today?
Buzz - Well to me, the first talk was the brilliant choice. So, I'm very glad about Andreas [Bausch] because he's brilliant. It was really showing if you take a thing we understand well, you take the machines which regulate cell shape - actin and myosin - two machines that we know exactly what they do. They've been studied for decades, we know exactly their molecular behaviour, their physical parameters. You put them together and you change the amount of filaments, you get behaviours that are extraordinary and Andreas doesn't understand how they arise, these beautiful things. It shows that simple rules can generate fabulous kind of behaviours.
In a way in biology, how can you reconcile these self-organising things that you have in tornadoes that we heard about - there's one in the Philippines this week, a devastating self-organising wind system - that's a bit like what is happening in the actin filaments. The biologists have said you have a genome and two identical twins are identical. So, biology is a lot about how the genome is taming and using self-organisation.
And so to me, that's a theme that runs across this tension between genes and self-organisation. The Mendel medal-winner Stan Leibler comes from physics and he just looks at the essence of things, and looking at things like hereditary and non-hereditary factors in determining organism behaviour and population, ecological behaviour - really new ways of thinking about complexity in life science and how much of it is just direct control like computer programmes, which is often where we used to think versus self-organisation. The mixture between the two is where I think life arises, which is I think is why the meeting is very alive, in fact.
Kat - What for you was the one thing that stood out that made you go, "Wow! That's fascinating! Or, that's weird!"?
Buzz - Well, when I heard about molecular biology which I work in and single gene research, you never get a wow factor because it doesn't mean anything. You find a gene that regulates even cancer. So, why that gene? What does it matter? But a lot of the biology here in this meeting is inspired by what is inspired by nature. Look at it and the forms are beautiful, the dynamics are almost inexplicable. I'm trying to tease apart those life processes in all these different talks and in almost every talk, that's why we've chosen people because they think that this is biology and I think that's what's - you know, all of it is amazing.
Life is amazing. Look at a hand or a leaf, or a seed, or anything. It's all pretty wow! That's why I'm a scientist. In the UK, at the moment, the public are interested in science and it's because it is wondrous. I think people don't feel that's excluded and I think in the audience too, we don't feel as excluded now. Physicists don't feel excluded from biology and biologists aren't excluded from using modelling. I think it's a nice time where we can all go "wow!" and then also stop and try to understand how things work which is hard, but that's what's fun. It's not demystifying. I think it doesn't take the magic out of the stars to understand them. It doesn't take the magic out of development. We don't have to worry about demystifying nature. We will never understand it, but we can get close and I enjoy that. I think the meeting showed a lot of people we're getting close, but also showing what is not known which is also clear in many talks.
Kat - We heard talks ranging from the shape to snap dragons to tiny molecules moving around, to the inner ear of zebra fish, all sorts of things. What would be your take home message from this meeting?
Buzz - I mean, in a way, it's partly we've invited people we like to hear and people we like to be up-to-date with. I think a lot of research exemplifies what I think is good science. Not all science is good. There's a lot of bad science, I would say and that 's mainly because it's hard. It's hard to do well and I think it's a bit of a lesson for me with all these talks and lessons how to do science, how to define a problem well, to setup an experimental system where it's tractable, dig deeper in understanding more and it can take 20 years. But you've got ways to really make progress.
We should all not sit down with the problem that we've been told to study. We should think about what's important about what's interesting in life and what we think is a fascinating problem. If we're going to spend our time night and day, thinking about things we should do something we think is a deep important problem, a beautiful problem and thinking about what's the best way to do it, not go to our catalogue and order what's easy, but think hard about let's find a tractable way to address a beautiful interesting problem.
Kat - That was Professor Buzz Baum from UCL. Congratulations to the winner of this year's Genetics Society Mendel Medal, Professor Stan Leibler, whose fascinating presentation about making mathematical models of complex biological systems we heard mentioned there. In his talk, he described how he and his team are taking small model ecosystems made up of bacteria, algae and single-celled animals, tracking them as they evolve, and trying to construct mathematical models that describe it. His work is helping to shed light on the processes and rules that underpin evolution, just as the medal's namesake, Mendel, did back in the 19th century.
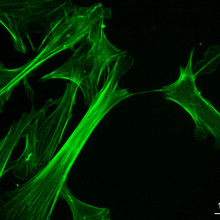
08:26 - Prof Andreas Bausch - Making shapes
Prof Andreas Bausch - Making shapes
with Prof Andreas Bausch, Technical University of Munich
Now let's hear more from one of the other talks Buzz mentioned, from Professor Andreas Bausch from the Technical University of Munich in Germany. He's trying to understand how patterns and structures form in nature, and showed some beautiful and striking movies of simple molecules coming together to create all kinds of shapes that move around. I asked him to explain how he and his team generated these stunning images from simple biological molecules.
Andreas - So, my model system is the cytoskeleton which is a polymer network inside of cells. We try to purify the components and then we see how they self-organise in beautiful spherical spirals or going to form waves, and so on. We try to really control what kind of patterns emerge.
Kat - Can you tell me a little bit more about the cytoskeleton? What is it inside cells?
Andreas - So, this is a polymer network which makes the cells stiff and gives us all the shapes.
Kat - It's almost like a scaffold inside the cell?
Andreas - Yeah, right. It's a scaffold and it's responsible for all kind of processes of cell division, cell migration, transport inside of cells. So, it's a rather universal polymer network and there's a lot of physics behind it. I think the beauty here is that a lot of physical concepts and measurement techniques are necessary to explore this kind of very complicated polymer network. It's much more complicated than all the polymer networks we know from a daily basis. So rubber is trivial compared to the complexity we have in cells. That's actually what is life about. It's already have high complexity and high integration density of functionality on this very small scale to have all these functions on this very small length scales.
Kat - So, what do you do in your lab to study these very, very complex systems?
Andreas - The idea is that we go from a bottom-up approach where we purify components and add step by step complexity and control the complexity which is kind of completely different than traditional biology would do it, that they come from a cell organism and go down. We try to really be on the physics approach where we really add the complexity step by step.
Kat - So, you're starting with the building blocks of a scaffold, putting them all in a test tube and seeing what happens.
Andreas - Yeah. Basically, that's what we do. We mix and watch.
Kat - We saw some beautiful, beautiful things. Tell me about some of the patterns you see.
Andreas - So, we see spirals, for example, where they move around or the galaxy forms where you get density waves in the spiral. You'll see waves like ocean waves walking over the cover slide. The amazing thing is that we span a lot of the length scale. So, the single filament is 7 nanometre and patterns are up to centimetres. So, this is unheard of length scales you're expanding here, where you get really kind of higher order structures out of very small interactions at very small length scales. Then we see also some shapes where we have spherical objects which change their shape into citrons or scallops or whatever. So, there's all kind of a zoo of different patterns and forms which we have here. That's the fascinating shapes are the fascinating thing here.
Kat - Sometimes it can be hard to think how do we go from the information amount of genes, it makes proteins and then it organises into these amazing structures. But looking at your images, you kind of start to see how life might work.
Andreas - Yeah, I guess we're on the upper end from the gene expression. We don't think about gene expression and the regulation of the gene expression. We have already the proteins. If you just let 2 or 3 proteins interact, it starts to emerge, the shapes emerge and patterns and dynamics emerge, and that's the fascinating thing here. The goal is evidently that we really want to mimic some real process like cell division or cell migration in the test tube with purified components. That's the ultimate goal we have here.
Kat - What's the weirdest thing you've seen in the results that you've got?
Andreas - The weirdest thing, I guess they all were weird the first time we saw them because they all were unexpected. The most fascinating I think these days is now, these vesicles which change shape in a very deterministic way which we never expected, in a very kind of mathematically pure, which nobody would expect it because it should unordered. So, we see kind of pure physics and pure mathematics coming out from a very complicated four-component system and that's kind of fascinating.
Kat - Some people say, biology, it's really just applied physics. Do you think we can break down the amazing process of life to physical rules one day?
Andreas - I guess that's exactly what's happening right now and I think we need much more physics coming into this complexity of biology to really break it down to principles and identify principles behind it. I think these experiments showed examples where you see that there is some hope that we can come up with some minimalistic systems where you see that already 3 or 4 components do a function. In nature, in real biology, you may have 10 or 20 proteins doing that, but four with defined function are enough to do it. And then you would have understood quite a bit already.
Kat - That was Professor Andreas Bausch from the Technical University of Munich.
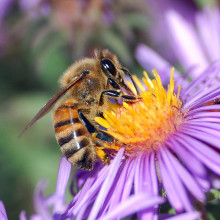
13:36 - Bee sex is complex
Bee sex is complex
Sex has just got a bit more complicated - for bees, at least. Researchers at the University of Cologne have discovered that the genes responsible for determining whether a bee is a queen, a female worker or a male drone are more complex than previously thought, publishing their findings in the journal Molecular Biology and Evolution.
Male drone honeybees hatch from eggs that have not been fertilised, while female workers come from fertilised ones. In addition, female workers inherit two different copies of a gene called csd, and these copies must be sufficiently different for the female bee to develop and thrive. Until now, there were thought to be about 20 different versions of csd, but now the researchers think there may be as many as 145 worldwide, which evolved in a relatively short period of time. The scientists think that the rapid production of this large number of variations helps to ensure a high level of genetic diversity in bee colonies, and could help to prevent inbreeding.
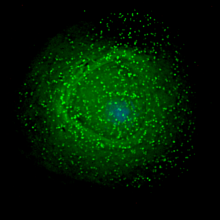
14:35 - Uncovering HIV resistance
Uncovering HIV resistance
Swiss scientists have made a step forward in understanding how resistance to HIV could be encoded within our own genes, according to a new paper in the journal eLife. Every person infected with HIV mounts some kind of immune response to the virus, and some people can even hold the infection at bay without taking anti-viral drugs. The researchers, led by Jacques Fellay, traced how HIV viruses sampled from more than a thousand infected people had genetically changed over time, in response to attacks by the immune system, looking at more than 3,000 mutations.
Then they also mapped the gene variations in the human genome that might be responsible for fighting back. Importantly, the researchers needed to look at data from samples taken before there were effective HIV treatments, to make sure they were only focusing on the natural effects of the immune system, so they had to trawl databases from the 1980s. It's the biggest global overview to date of the genes involved in HIV resistance, and the scientists think that their results could one day lead to more effective, genetically personalised therapies.
15:39 - Fountain-Of-Youth Gene
Fountain-Of-Youth Gene
It's long been known that young animals recover more quickly from injuries than older ones - from insects and fish to mammals including ourselves. But what's not known is why or how this happens. Now researchers from Boston Children's Hospital and Harvard Medical School in the US have found a gene that seems to be responsible, publishing their findings in the journal Cell.
The researchers suspected that a gene called Lin28a, which is very active in embryos from many species, but shut down in adults, may be playing a role in the phenomenon. To find out, they reactivated the gene in adult mice, and discovered that these animals could grow their hair back faster after shaving and had quicker wound healing.
Lin28a is involved in activating processes involved in energy production and use within cells, known as metabolism, and the researchers found they could bypass the need to switch the gene on by using drugs that directly stimulate cell metabolism. The scientists speculate that Lin28a - or drugs that work in a similar way to it - could form part of a "healing cocktail" that could help restore youthful healing properties to older adults, potentially opening up exciting possibilities for fixing injuries, healing wounds, or treating and diseases.
16:51 - Gene linked to fungal infections
Gene linked to fungal infections
If you've ever had athlete's foot, you've had a fungal infection. Such infections are common and easily cleared up with over-the-counter anti-fungal drugs. But in rare cases, such usually harmless fungal infections can get beneath the skin and spread to the bones, gut, immune system and even the brain, causing a condition called deep dermatophytosis which can be fatal.
Writing in the New England Journal of Medicine, scientists at The Rockefeller University in the US and Necker Medical School in Paris have now discovered a gene fault that allows the fungus to spread in this way. To find the faulty gene, called CARD9, the researchers looked at DNA from 17 people who had succumbed to deep dermatophytosis but were otherwise healthy, with normal functioning immune systems. Previous research had suggested CARD9 might be the suspect, and the scientists found it was faulty in all 17 of the patients.
The scientists think their finding explains why fungal infections sometimes spread into the body, and why they can be so hard to treat when they do. In the case of families that carry the gene fault, this could lead to genetic testing, as well as prevention advice and targeted treatments. And it also lends weight to the idea that genetic variations between people make the difference between some people being able to shrug off minor infections while others fall seriously ill.
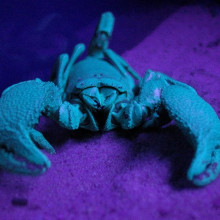
18:10 - Mice resist scorpion stings
Mice resist scorpion stings
Researchers led by Ashlee Rowe in the US have reported that little grasshopper mice are resistant to the painful effects of the highly toxic venom of bark scorpions, thanks to a genetic quirk. Bark scorpion stings can kill other mammals of similar size, yet it seems to act as a painkiller in grasshopper mice, rather than a toxic poison. Writing in the journal Science, the researchers went into the desert to collect mice and scorpions, testing the effects of injecting either scorpion toxin or salt water placebo into the animal's paws. But rather than licking their paws more in response to the toxin - the usual pain response for these mice - they licked them less than when injected with salt water.
By looking at the gene sequences for the receptor molecules that respond to the toxin, the researchers discovered a single crucial difference between the receptors in the grasshopper mice and those in other mammals that do feel pain from the scorpion's stings. The change is enough to turn the toxin from a pain-causer to a painkiller. The scientists still don't know why the grasshopper mice don't get poisoned by the stings, but they do hope their discovery could lead to ways to better develop painkillers for humans, rather than mice.
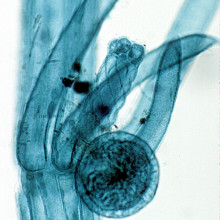
19:32 - Prof Ray Goldstein - Streaming in cells
Prof Ray Goldstein - Streaming in cells
with Prof Ray Goldstein - Cambridge University
Now it's time to hear more from the Genetics Society Autumn Meeting, from Genes to Shape. Professor Ray Goldstein, from the University of Cambridge, studies how molecules organise themselves inside cells, focusing in particular on plant-like algae called Chara. I asked him to describe to me what you can see inside the surprisingly large cells of these plants if you look down a microscope.
Ray - You'd see a beautiful stately flow of about up to a tenth of a millimetre per second. Sounds ridiculously slow, but by biological standards, that's fast. In a kind of barber pole arrangement, two helical bands of flow, one going up the cell, one going down the cell in this beautiful regular pattern. You'd see various organelles within the cell being carried along by this streaming. It's driven by the action of motor proteins that walk along filaments. So for instance, myosin walking along actin or kinesin walking along microtubules, and carrying cargo one store to another, the action of moving that cargo entrains fluid. And because there's some kind of long range order in the filaments, one sees ordered pattern of one degree or another in the fluid flow.
Kat - So, we're not talking about just fluids sloshing about inside a big cell. This is a very, very organised system.
Ray - Well, there are two basic types. There are those that are super highly organised, regular geometric structures and there are others that are more disordered such as in the oocyte of a fruit fly which is one commonly studied situation. But even there, it's not completely disordered. The microtubules that drive it are organised from the cell periphery and although it looks a bit turbulent or chaotic to the eye, there is structure in it anyway. So, it is a structured flow to some degree enough.
Kat - So, what's the question that your work is trying to answer?
Ray - Our work on cytoplasmic streaming is fundamentally aimed in understanding its role in biology. There's been a lot of work over the centuries in visualising it and quantifying it, but there's still not a clear indication of its purpose. Is it to mix the contents of the cell, is it a by-product of a transport process? It's very unclear. So, we're trying to unravel this step by step.
Kat - So, you can almost imagine this little army of proteins marching things around the cell. How do you study them and how do you tell what they're up to?
Ray - Well, the first thing is, one can visualise the flow, independent of the driving force, by having tracer particles of one sense or another. So, they give you a direct readout of what the fluid flow is doing. But it's also possible to label the various agents going on here and actually to have a direct visualisation in one sense or another of the cargo.
Kat - The fascinating thing about these kind of systems - the flows and all these things - is that they are completely directed within the cell by itself. They're self-organising. What are the clues that we have so far about how these work?
Ray - Well, I would say that in the case of large plant cells, which is the one that I've been focusing on most, there are tantalising experimental observations that show that you can disrupt the underlying network of the filaments. When these chemicals that do get disruption are removed, the system can heal itself and reform the pattern of streaming, perhaps with a different precise origin and all of that, but it's the same basic pattern just shifted around a bit. That already speaks to self-organisation.
We also know that as some of these cells grow, the pattern changes in a systematic way and this suggests that there's a very clear direction going on, but again, this further indicates a self-organisation process. In the case of the more disordered flows, we have changes that occur during development that really speak to self-organisation processes, some chemical that was present in large quantities at this point in time disappears and then all of a sudden, a new pattern emerges.
Kat - And how widely across biology do we see these properties of self-organisation at this kind of level happening?
Ray - Well, without being too clichéd, I think that it's fair to say that self organisation occurs on length scales ranging from a few microns to a few kilometres. You can look at wildebeast on the plains of the Serengeti and you can see self-organisation into coherent locomotion. You can see that in locust swarms, you can see it in bacteria in a Petri dish and you can see it inside a single cell. Now, it's wrong to just imagine that there's some single underlying mechanism crossing all those length scales, but the mathematical structure of a theory that would explain it can have some commonality.
Kat - And how close are we to coming up with these kind of theories or is it just very complicated?
Ray - I think we're actually fairly close because certain model systems allow us to make measurements to test in detail the predictions of a theory. And so, we can apply what's known as the scientific method. Here we are in Royal Society where Newton was so prominent and it's thanks to him we really use the scientific method. We can use that and go back and forth. We can do an experiment in a way that was not possible 10 or 15 years ago thanks to technological advances of imaging and microscopy.
Kat - Some of the work that we've heard about today, it's looking at very specialised model systems, very rarefied things, or single cells. Do you think one day we will be able to describe the organisation for example of an embryo, a human embryo as it goes from one cell to many and all the things that move around there?
Ray - I certainly think we all believe so. We tend to be very reductionist so we start with simpler things where we think we'd have more capacity to understand all that's going on. But it's remarkable how much progress has been made. Mostly, it's a question of courage I think. We just have to sort of launch down the path and try to figure out what it is we need to measure and what a mathematical model would look like and how to test it and go back and forth.
Kat - For you, what are the questions that you still really want to answer? What are the known unknowns for you?
Ray - A known unknown would be basically the question of the purpose of streaming. Of course, it may not have a single purpose, but basically, why have cells engineered these large scale flows? Are the flows themselves just by-products of a transport process or do they play a particular role in the self-organisation that happens? This is often the case, it appears to be in the development of the body plan of higher organisms. We'd really like to have a connection between the microscopic action of the motor proteins that go whizzing along these filaments and the flows that we see. It's not a very straightforward connection because fluid mechanics is complicated and the micro scales are hard to see. But those are the burning questions.
Kat - That was Professor Ray Goldstein from Cambridge University.
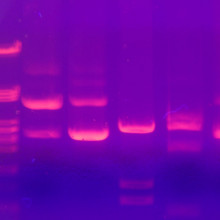
Could you have a non-contact DNA scanner?
Listener Liam asks "Could you ever use some kind of scanner to scan someone for their DNA without physically touching them?" To answer, here's DJ de Koning, who's a professor at the Swedish University of Agricultural Sciences.
DJ - As far as I can imagine and look into the future, I think we will always need some form of sample from the person or organism we're trying to determine a genotype or DNA sequence for. But we need very little material these days and I think all of us leave a lot of our DNA lying around without realising it, from which the DNA could be isolated because we only need a single cell. So, we can isolate DNA from a razor, a comb, a toilet sample, you name it. There's probably quite a bit of your DNA around you where you have been sitting or where you have been blowing your nose, something like that. So, it may not need contact or conscious sample taking, but you do need something that was once part of you to do DNA work.
Kat - Thanks to DJ de Koning, and also our listener Liam. And if you've got any questions about genes, DNA and genetics you'd like us to answer, just email them to me at genetics@thenakedscientists.com.
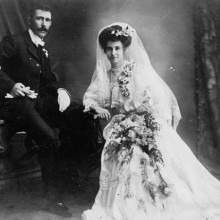
27:50 - Gene of the month - Matrimony
Gene of the month - Matrimony
with Kat Arney
And finally, our gene of the month is in search of the perfect union - it's called Matrimony. Found in fruit flies, the protein made from the Matrimony gene is stockpiled in female flies' egg cells, known as oocytes, together with another protein called cortex. It's responsible for holding together the chromosomes in the egg cells until they're ready to divide when the egg gets fertilised. The protein quickly vanishes once fertilisation happens, as it gets broken down and destroyed. Fly eggs with excessive levels of Matrimony don't develop properly, suggesting that the getting the right levels of the protein is essential for making healthy new flies.
If you're feeling less like a happy marriage, then you could always turn to the gene named after one of the unluckier wives in history. The biblical character Lot's wife is supposed to have turned back to look at her hometown of Sodom as she was fleeing from its destruction, and was turned into a pillar of salt. The fruit fly gene Lot's Wife, also known as Drop Dead, is responsible for a range of jobs including how the animals respond to very salty conditions.
- Previous Restore, repair, retain!
- Next What is opium
Comments
Add a comment