On the Flip Side - Earth's Magnetic Field
This week we’re looking at the magnetic field keeping our planet safe, finding out how it's generated and whether some animals can actually see it. Plus, news of a technique to read out the time of our body clocks, the people making the case to reinstate Pluto as a planet, and how red alert signals can spread through plants in just seconds after something starts to eat them.
In this episode

00:56 - Time-telling tissues
Time-telling tissues
with Dr Rosemary Braun, Northwestern University
As anyone who has ever suffered from jetlag knows, we are indeed all slaves to the rhythm - in this case our circadian rhythms - or body clocks. And the reason we feel so ghastly when our internal clocks go off kilter is because every cell in our bodies uses time to control what it does and when. Tissues grow and repair themselves at certain times of the day; our metabolism changes dramatically between dawn and dusk. And medicines and vaccines given at one time of day can be much more effective than the same drug administered just hours later. This means that there is enormous potential to improve healthcare - but only if we can reliably tell what time our tissues think it is.
Now Rosemary Braun, from Northwestern University in the US, has developed a way to do this by comparing the activities of a collection of different genes in blood cells. Chris Smith spoke to Rosemary...
Rosemary - You have an internal clock in your body. The signal for it originates in your brain but it orchestrates a wide variety of processes across your body including when you feel sleepy; it regulates your digestion; it regulates your blood pressure to get you ready for the day; it regulates your body temperature to allow you to sleep comfortably at night. And all of these things are coordinated by clocks that exist in each and every cell of your bodies. There’s a master clock in your brain and it synchronizes all of these little tiny cellular clocks.
Chris - Do we know, Rosemary, what the clockwork is inside all these cells that are running these clocks to keep time like that?
Rosemary - Yeah. This is really fascinating. It’s a set of genes that have an activity that varies over the course of the day and they interact with each other in a little circuit that allows them to regulate each other. So one comes up, it pushes another one down, and this push and pull results in an ebb and flow of activity with a 24 hour cycle.
Chris - And what, the brain centre then sets the tone for the rest of the body how?
Rosemary - Your brain secretes hormones that your cells pick up in order to reset their clocks so that they’re right in sync with what your brain is telling them.
Chris - So in theory then, if I were to read one of the clock signals from the end of my little finger it should be telling the same time if all is well as my brain?
Rosemary - Exactly.
Chris - So why does this matter? Why do we need clocks in my finger if I have one in my brain at all?
Rosemary - The reason that this is so important is that you need all of the processes across your body to be orchestrated in sync so that you remain healthy. Given that it controls things ranging from not just sleep to digestion and blood pressure, you can imagine that it has an enormous impact on your health if it’s misaligned in some way. In fact, research has shown that circadian misalignment, when your clock is out of sync with your environment, is tied to diseases ranging from depression to diabetes, heart disease, Alzheimer’s, so having a misaligned clock can really adversely impact your health.
Chris - Is that a part of our clinical practice? So when we go and seek treatments are they aligned with our clock to make sure that we’re doing the right thing at the right time in the body’s clock cycle?
Rosemary - Presently they’re not and that’s what we’re trying to change. We know that it’s incredibly important but right now it’s very difficult to measure it. The current way that people have been measuring people’s internal physiological clocks is by taking samples every hour across the day and night. And you can imagine that’s not really something that most people would want to do, so we set out to develop and easy blood test so that we can monitor people’s circadian health and use that to make treatment decisions.
Chris - When you say it’s a blood test, what are you measuring?
Rosemary - It takes two blood samples: say one in the morning, one in the evening. They can actually be taken at any time of day as long as they’re separated by a few hours, and then we look for the activity of different genes in the blood. So there aren’t just the core clock genes that are responding to the 24 hour rhythm. The clock actually controls a huge number of other genes that sort of move in sync with the clock and those are the markers that we’re looking for in the blood.
Chris - So you take some blood, what do you get blood cells out of the blood and then look in those living blood cells to see what the genes levels are?
Rosemary - Yeah, exactly.
Chris - How many genes do you consider then in order to get a readout like this?
Rosemary - We started out our research by looking at all of the genes that we could measure - that’s about 20 thousand different genes, and we used a pretty sophisticated computational algorithm to try to whittle that down to a manageable amount. And what our algorithms told us was that there is a set of about 41 genes that change over the course of the day, and by looking a the levels of those 41 genes we can pinpoint the time in your body.
Chris - Right. So you literally are saying that we know that when it’s 7 o’clock by my body clock this gene should be doing this and it’s counterpart should be doing this, and because you know what the relationships are across that small cluster of genes you’ve got a reasonably accurate way of predicting my body clock time?
Rosemary - That’s right. And it’s accurate to within about an hour and a half which is good enough to be able to then make treatment decisions based on it.
Chris - What sort of a difference will it make then?
Rosemary - Drugs have a different effectiveness depending on where they are taken. This is well known for certain blood pressure drugs and chemotherapies that they’re differentially affective at different times of day. But the optimal time for me to take my blood pressure drug might be different from the optimal time for you to take your blood pressure drug. If we can measure the time in your body, we can tell you exactly when the optimal time is for you to take your medicines. That means we could use lower doses, reduce the risk of side effects, and hopefully have more effective treatments.
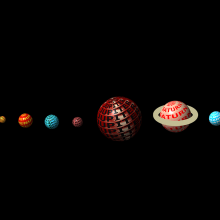
06:59 - The Pluto debate: planet or dwarf planet?
The Pluto debate: planet or dwarf planet?
Are you a Pluto sympathiser who’s been made despondent by this miniature marble’s demotion to a “dwarf planet” a little while ago? A paper has recently been published arguing that Pluto should in fact be reclassified as a planet again. But why? And why was it “dwarfed” in the first place? Georgia Mills and Adam Murphy have been doing a bit of star gazing to find out...
Georgia - So back when you and I were kids, it was very easy to remember the order of the planets in our solar system. My very easy method just speeds up naming planets - Mercury, Venus, Earth, Mars, Jupiter, Saturn, Uranus, Neptune and Pluto.
Adam - Yeah. Except your very easy method got it wrong. In 2006, the International Astronomical Union voted to reclassify Pluto as a dwarf planet.
Georgia - #justiceforpluto. So why did they feel the need to debate this?
Adam - Back in 1930 when Pluto was discovered it was the only celestial body in that part of the sky that we knew about. But there are actually a lot of bodies out there on the fringes of our solar system.
Georgia - Ah, yes. This is the Kuiper Belt. This is the massive doughnut on the outer solar system which is full of icy and gassy bodies.
Adam - Exactly. And that’s the problem. Pluto is just one of many objects inhabiting the same orbital zone and we’ve been finding things there almost as big as Pluto. One called Quaoar was found found in 2002, Sedna in 2003, and Eris in 2005, which was actually bigger than Pluto.
Georgia - Ah, 2005. The year before the big debate!
Adam - Yeah, exactly. Did we have shed loads of new planets all of a sudden?
Georgia - You’d need a very long mnemonic.
Adam - Something had to be done so a committee sat down in Prague to decide what constitutes a planet:
After a lot of debating they had a vote on the following definition: a planet is a celestial body which a) is in orbit around the Sun...
Georgia - Check.
Adam - b) has sufficient mass for its self-gravity to overcome rigid body forces so that it assumes a hydrostatic equilibrium shape...
Georgia - Uhh!
Adam - Is it round?
Georgia - Check.
Adam - and c) has it cleared the neighbourhood around its orbit? That is to say, is it dominant enough in its orbit that anything else around has either been absorbed or booted out into space?
Georgia - Ah, failed on the last hurdle. The Kuiper Belt is a very busy neighbourhood.
Adam - Exactly. So under that definition Pluto gets booted from the ‘exclusive planetary club’ into the ‘dwarf planet economy lounge.’
Georgia - Hang on. Are we sure that the other planets fulfill that last definition? There’s a heck of a lot of junk flying around the rest of our solar system.
Adam - Well, some have argued that under the new definition, Earth and Jupiter fail to meet the IAUs definition but they’re in the minority.
Georgia - I hope Earth gets to stay a planet. I don’t know how I’d feel about living on a non-planet. What about this new paper? They’re definitely team “Pluto is a planet”.
Adam - Very much so. Lots have argued that Pluto should be reinstated since the decision for a bunch of different reasons. This time they’ve gone through the literature and look for examples about the third part of the definition actually being used.
Georgia - Ah. This is the one about being the most dominant in the orbit?
Adam - That’s the one. They checked in papers in the past 200 years.
Georgia - They really care about Pluto.
Adam - And they found it’s barely been historically - only once in the the 19th century. They argue that it’s an arbitrary definition.
Georgia - Isn’t that the point of a definition?
Adam - Well, it’s hardly the first or the strongest challenge to the ruling and it won’t be the last. But the IAU are reportedly happy to debate the topic again so we’ll have to watch this… Space.
Georgia - I’m demoting you from ‘human’ for that!
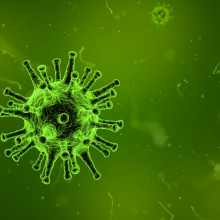
10:37 - What do viruses do inside cells?
What do viruses do inside cells?
with Omer Ziv - Cambridge University
As we head into winter in the Northern Hemisphere, as well as long nights and dreary weather, another certainty is that - soon - we’ll all be succumbing to this season’s circulating strains of colds and flu viruses. At the moment, we can’t do much about them - except treat the symptoms - because we don’t know the details of what going on when a virus gets into one of our cells. So finding viral “Achilles’ heels” that we can hit with drugs to trip up an infection is very tricky. Now though, Cambridge scientist Omer Ziv has found a cunning way to freeze an infecting virus in its tracks and then pull out the parts of the cell that the virus is interacting with so we can discover how it makes us ill and possibly where to focus our drug-developing activities. He spoke with Chris Smith...
Omer - We are interested in viruses. Viruses are those little creatures that go inside our bodies and make us ill. And we are interest to know how those viruses manipulate our cells, practically tell the cell: “stop everything you’ve been doing so far and start making more viruses”.
Chris - Yeah. Cause viruses are sort of like the pirates of the microbial world, aren’t they? They have to hijack our cells and turn them into virus factories because they’re so tiny they don’t have space inside the virus particle for any of the machinery that you need to make new viruses. They need one our cells to do that.
Omer - Yes, exactly. They enter our cells and manipulate whatever the cell is doing but we don’t know, essentially, how.
Chris - What have you therefore invented here? How does your technique shed light on that?
Omer - We’ve been developing a technique that enables us to freeze in time the virus infection and find out how viruses interact with the host on the molecular level.
Chris - Is that a bit like if I were to put a virus into a cell, wait a little while and then, as you say, freeze time and then look inside the cell and ask what bits of the virus are binding onto, or interacting with, or controlling what bits of the cell? So I can see what’s having a chemical conversation with what.
Omer - Yes, exactly. And once we find those interactions, assuming that part of them might be essential for the virus, we can think then of finding ways to target, to inhibit, interfere with those interactions and affect the virus life cycle.
Chris - How have you done this though? How do you do that freezing in time effect?
Omer - To do that we need to glue the interacting molecules together to fix those interactions and then extract information. We used small chemicals to enable us to link, to glue those interactions together and identify the interacting partners.
Chris - How do you make the glue set? How do you actually say right, now I want to freeze time and make that binding effect kick in?
Omer - The glueing starts whenever I treat the infected cells with those small chemicals that can enter the cell and glue physically the interacting molecules one to each other.
Chris - Are you saying then that if you can spot what these interactions are it might highlight to us, much more quickly than we would otherwise be able to discover them, potential, essential processes that the virus relies on to grow and make us unwell? And, therefore, you could engineer some way of either switching off that target or putting something into the cell that would stop that interaction and, therefore, it could block the virus?
Omer - Yes, exactly. We are interested both in the biology so the new technique might teach us how this virus has replicated inside the cells, and also understand whether those interactions are targetable and whether we use them for our development of new mediations.
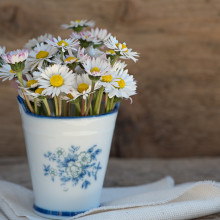
15:05 - How does a plant sound the alarm?
How does a plant sound the alarm?
with Dr Phil Wigge, Sainsbury Laboratory, Cambridge University
If something starts to eat us humans, we can register our displeasure by either making a fast getaway or beating off the guilty party. But plants don’t have this luxury – they’re literally rooted to the spot so they need to resort to other means to discourage things from eating them. One deterrent at their disposal is to make themselves taste unappealing. But they don’t want to waste resources on tasting bad until they need to, so how do they send a rapid ‘I’m being eaten’ message all around the plant? A recent paper in the journal Science, reveals the answer. Georgia Mills paid a visit to the University of Cambridge’s Sainsbury lab, to speak to Philip Wigge, who wasn’t involved directly in the study, but he does work on how plants can sense their environment.
Georgia - It looks like a sort of bank vault in here.
Philip - This is where we grow our plants you see. This is a plant called Arabidopsis and it’s just a small mustard plant. And this was the same plant that was used in the study which is described in this paper. You can see it’s quite a modest looking plant - it’s got small white leaves but for research it’s been an absolute boon. This is really sort of the lab mouse for plant research because it has a fully sequenced genome and it’s very well understood.
Georgia - But, unfortunately for us sequenced genome or no, Arabidopsis also lives in an incredibly noisy home. So we went to find a quieter part of the greenhouse…
Philip - This is what I thought was a quiet area.
Georgia - Which was harder than it sounds.
Philip - This is quite noisy too. There are a few ambient noises that you don’t think about when you’re working here.
Georgia - Ah, sweet silence. We settled down to chat amongst some other plant specimens who, believe it or not, may have felt our presence. And they need to…
Philip - You can imagine, if you are a delicious plant sitting outside trying to grow in the sunshine, and if an army of caterpillars comes along you really want to be able to respond very quickly and defend yourself as best as you can. And that’s quite complex because obviously you can’t move away, so plants have to be very resourceful and very perceptive when dealing with pests like caterpillars that want to have them for lunch.
So if you imagine this plant here, you could imagine this leaf, and if we just pull the leaf apart. And as I do that what I’m doing is I’m pulling apart millions of cells, so millions of cells are being crushed and broken open. Now it turns out, although we can’t see it, as we tear this leaf the plant is actually responding inside within seconds.
Georgia - We can’t see it, but we have known that plants are able to do something like this for a long time. But, unlike you and me, they don’t have the luxury of a central nervous system, so this group, among many others, were wondering how are they sending these messages?
Philip - What they show is that the plant is using a small amino acid called glutamate. Now glutamate is also used in humans as a neurotransmitter remarkably enough. So what happens when a cell is damaged is that the cell releases glutamate into the open, and that glutamate is then sensed and picked up by channels. And when these channels become activated they release calcium, and it’s the calcium signal that this paper shows, is a mobile signal that travels within seconds throughout the plant. And then what the plant does is it activates the expression of genes that control a response to pathogens. So one way for a plant to protect against the caterpillar is to make itself taste very bad.
Georgia - How did they find this out? How did they work out this is what was going on inside the plant?
Philip - They used a number of tools from molecular biology to identify the actual receptors. They had a hypothesis that calcium could be involved and that glutamate could be the signal and then they were able to find particular plants that lack the channels that respond to glutamate. What they were then able to show was that if you take away just these channels within the plant, the plant no longer transmits this signal. That’s kind of definitive proof, that you need these channels in order to respond to herbivory.
Georgia - I feel kind of bad now for pulling up daisies to make daisy chains when they’re sending out these ‘bah help me’ signals.
Philip - That’s a good point actually. We know plants respond very much to any kind of perturbation and they have these large scale changes. Whether or not they’re aware of this or whether they feel pain is probably unlikely, so I wouldn’t feel too guilty about cutting the lawn. But it’s interesting to be aware when you do but the lawn you’re causing these large scale changes in how every single blade of grass is responding to being cut.
Georgia - And you get that lovely smell. Is that their death throes?
Philip - That’s one way of looking at it but I try not to think about that.
Georgia - As someone who works on this, in this field how important and how much of a change has this paper brought would you say?
Philip - Oh, I think it’s a great study and it’ll go into the textbooks I think. There’s a whole range of very fundamental questions that we still don’t know about plants. This is quite remarkable in a way because we are so dependent on farm grown plants to sustain the entire human population. And so until relatively recently farming has been largely a sort of trial and error process and just in the last few decades we’ve started to understand the molecular basis by which plants grow and how they develop. And what this means is that it enables us to potentially improve crop yields and food security.
There’s no question that herbivory is a major problem in many areas of agriculture, so if we can create smarter plants that are more perceptive and more resilient to feeding insects then that’s going to be, potentially, a huge advantage.
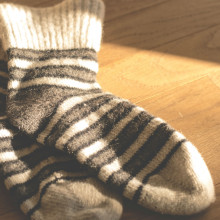
20:55 - Mosquito-repelling socks
Mosquito-repelling socks
with Dr Mthokozisi Sibanda, University of Pretoria
Here’s a story about something that you’re definitely not going to see on the catwalk at London Fashion Week (it’s something highly practical for a change): socks that could save you from mosquito bites and also malaria. Chris Smith spoke with Mthokozisi Sibanda from the University of Pretoria.
Mthokozisi - My name is Dr Mthokozisi Sibanda. I’m from the University of Pretoria.
When you’re outdoors mosquitoes will bite you on the ankles and feet 93 percent of the time. They are attracted by foot odour.
Chris - Isn’t that where we spray the insect repellent then?
Mthokozisi - Yes. You can spray topical insect repellent but it will quickly evaporate - in an hour or two it’s no longer effective, then you have to respray again. So we needed to come up with a way of having a long lasting formulation, so basically what we developed is a slow release technology. We spin a fibre and the fibre is specially engineered to hold a liquid insect repellent and the fibre will slowly release that repellent over a long period of time. It can last up to eight months or if you knit the fibre into a textile you can wash that textile for a minimum 25 times and it’s still effective.
Chris - So you would put the formulation into this fibre using the technology you’ve invented and what, you then spin it into a pair of socks or an anklet or something that a person would wear?
Mthokozisi - That’s correct.
Chris - Does it work?
Mthokozisi - It works. We have tested it; we have actually published the results in a high impact scientific journal.
Chris - How effective is it and how did you do that testing?
Mthokozisi - Okay. There are a number of tests recommended by the World Health Organisation. The one that we use is a pretty agressive one where we put our formulated sock on one foot and a control sock, which is untreated, on the other foot. We put both feet in a cage with 300 female hungry mosquitoes. They have to make a choice: which ankle do they feed on. If you see all of them going to the untreated sock then you know that your sock is working.
Chris - That sounds like a really painful experiment. Did you do it?
Mthokozisi - I did it. But you know you have people who run these insectories and they use their arms to feed the mosquitoes. So what I did is really nothing to what other people do.
Chris - Things that people will do for science! But tell me about the technology that’s enabled you to do this? How does this clever fibre and fibre spinning technique work and how do you get the insect repellent in there in the first place?
Mthokozisi - What we make is what we call a bi-component fibre. One polymer is in the core and that polymer can absorb a high amount of oil. And we have a polymer that forms a shield around the core polymer so the oil will have to diffuse through that shield. In that way, the shield slows down the evaporation of the oil overall. If you knit the fibre into a textile and wash that textile, you only wash the outside of the fibre, the bulk of the liquid is still stored inside the fibre. It will still migrate to the surface and that’s how it’s replenished.
Chris - In essence, it’s a tube within a tube. And the tube inside the tube loves oily things, the tube outside the tube hates oily things. So you’ve got your repellent which is oily based in the middle and it’s obviously facing a barrier to diffuse out very slowly. What is the chemical that you’re using as the repellent - is that just DEET or something? Because that’s the industry leading standard DEET, isn’t it?
Mthokozisi - Yes. DEET is the standard repellent on the market right now. It’s got a bad reputation, unfortunately, although it has not been proven to be bad scientifically. So we are using an alternative repellent called IR3535 - it’s as good as Deet. We also use a natural repellent based on the eucalyptus tree.
Chris - Are these socks or whatever you design fashionable because obviously, especially with youngsters, people are not going to wear something that looks like a fashion disaster? So can you add colour, add patterning; make it like it’s a normal piece of everyday wear so it doesn’t look out of place?
Mthokozisi - Yeah. When it comes to that it is so easy because we can make the fibre into any colour - we just add a pigment. We can make any sock design. We actually combine our yarn with the liquid, with cotton wool to make it nice and comfortable and you can make a nice fashionable sock.
Chris - How will I know though when my socks are no longer working? Because it’s brilliant while it is working of, course, but there has to be a way of knowing when I’m no longer protected because otherwise I could be out there with false confidence and catch something.
Mthokozisi - Basically, we do lab testing to determine the length that these products can work, and we can sort of calculate the minimum length that you should keep them for. So they will be labeled to say they can work for this long and after this it’s recommended that you get a new product.
Chris - Can you recharge them or do you have to throw them away because, obviously, that’s not great from a sustainability point of view?
Mthokozisi - No, you’re right. You can’t recharge them and what we are doing right now is we are developing the fibre based on biodegradable polymer. There is research that is ongoing to use biodegradable polymer so that they can be disposable.
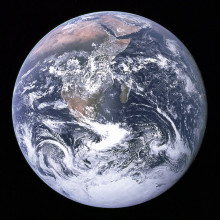
Earth's magnetic field
with Professor Kathy Whaler - The University of Edinburgh
Why is our planet magnetic? Have we always had the same magnetic field, and is it true that some animals can detect it and use it to find their way around? This week we’re going to try and find out. And with us to answer some of those questions is Kathy Whaler, from the University of Edinburgh. Kathy maps the Earth’s magnetic field, and she spoke to Chris Smith. First of all, Chris wanted to find out more about the asteroid name after her!
Kathy - When I was president of the Royal Astronomical Society, which also covers geophysics, I was awarded that at the end of my service.
Chris - What’s it called? Has it got a good name?
Kathy - It’s called Kathy Whaler. Very uninteresting I’m afraid. It sits in the asteroid belt.
Chris - An imaginative bunch these astronomers aren't they?
Good to have you with us Kathy. I’ve got a compass sitting here and when I go walking I use this to find my way around. It’s a small magnet which senses the planet’s magnetic field, responds to it, and tells me when I’m going in the right direction.
Why has the Earth got the ability to make a magnetic field and how’s it do it?
Kathy - We all sit and walk about and drive over the Earth’s crust, which is a brittle layer 7 to 60 kilometres thick depending on where you are - thinner over the oceans. And that’s made of silicon, of rocks and beneath that it’s also another rocky layer, the mantle. Slightly different in that it’s able to creep on rather slow timescales. So that’s the top half of the Earth. And when you get about halfway down there’s a real major change, so we go from silica dominated material to iron dominated material and the iron is where we get our magnetic field from. We get it specifically from the outer part of what we call the core, the area towards the centre of the Earth divided into a liquid outer part and a solid inner part.
Chris - Which of those two bits: the liquid outer part and the more solid inner part is responsible for making the magnetism?
Kathy - It’s the outer part because that undergoes a process we call convection. Basically it churns around and as that liquid moves then it’s able to self-generate a magnetic field.
Chris - Now given that this is going on thousands of kilometres beneath our feet, how do you, as a group of geologists, know that what you’ve told me is true?
Kathy - Well, we measure the field on different timescales by different mechanisms and that enables us to see the changes in the field, and the changes tell us that we have to have some active dynamic process that’s responsible for its generation. It’s not a residual field from when the Earth was formed, for instance or anything like that.
Chris - It has to be originating from that mobile core?
Kathy - It does.
Chris - Given that the Earth is losing heat all the time, Kathy, and cooling off, that includes the core doesn’t it? So if the core were to go solid, what would happen to our field?
Kathy - Well, if the core was to freeze out altogether then we would actually lose our magnetic field, and then we’d lose this protective shield that we’ve just been talking about that protects us from solar wind particles and things like that.
For instance, if you think about our near neighbour Mars, Mars used to have a magnetic field but it stopped being generated and, as a result, Mars lost its atmosphere.

How do magnets work?
with Dave Ansell, The Naked Scientists
What actually is a magnet? How do they work? Izzie Clarke met up with science demo king Dave Ansell to find out...
Izzie - Hello, hello. Let’s talk magnets. We’re here looking at magnets in this amazing workshop. I’m quite overwhelmed with all of the experiments we could do. What is a permanent magnet?
Dave - Let’s start off by looking in a really really small scale. Some materials, like iron is one of them, have atoms with more electrons going one way round than the other, and that creates an electric current which makes a little tiny electromagnet. Now on its own this doesn’t make something even magnetic. These little atoms will be randomly arranged and the magnetism will average out to zero.
But with some of these materials like iron, nickel and cobalt, for weird quantum mechanical reasons, these little tiny atomic magnets line up forming great big areas called domains with all of the magnets lined up. So all the magnetic fields add together so they can interact with things magnetically really strongly.
Izzie - Okay. So it’s like they’re all acting in one direction so overall they have this sort of magnetic pull essentially?
Dave - That’s right. And then if you put them near another magnet, all those magnets will interact and they’ll either stick or repel.
Izzie - Okay. How is it that something can stick to a magnet but it isn’t a magnet itself?
Dave - This goes back to the domains I was talking about earlier which are the small magnets inside a piece of iron. I’ve got a little metal nut here and inside it there are lots of magnetic domains and they’re all magnets themselves but they’re are arranged randomly so overall, it’s not a magnet. But if you put it near a magnet those domains will all twist round and line up so it becomes a magnet aligned with the original magnet, so a north pole is next to the south pole so it’s sticks. And we can see that because if we take a second nut it will stick to the first one, and a third nut will stick to that, and a fourth, fifth, sixth.
Izzie - So what we’ve got here is lots and lots of tiny little metal nuts. On their own they’re not stuck to each other; whereas you bring a magnet into the game and all of a sudden one sticks onto the magnet. Then you can stick another on the one nut and so on and you’ve got an even bigger magnet essentially?
Dave - That’s right. And with a material like iron, if you take it away from a magnet the magnetic domains just kind of randomise again and it stops being a magnet overall so I can take them off and they don’t stick to each other.
Izzie - There’s a big difference between something being a magnet and something having magnetic properties. Take your fridge for example: you can stick magnets on it therefore it has magnetic properties, but it’s not a giant magnet itself. I mean, it would make taking cutlery out of a drawer rather problematic, and these iron nuts are the same. On their own they don’t stick to each other but because they have magnetic properties as long as there’s a magnet in the vicinity they’ll stick and act like an extension of it.
But could we take one of these nuts and actually transform it into a permanent magnet itself?
Dave - First of all we need to start with the right material. You want something which is really hard to twist these domains round, and one of the best materials for that is neodymium iron boron, which is an alloy of all those three elements.
This is a lump of neodymium iron boron, and it will stick to a magnet but very weakly because not many of those domains are twisting round so it forms a very very weak magnet.
Izzie - Okay. So how would we turn that into a magnet?
Dave - What you have to do is make it easier to turn these domains round and the way you do that is by heating it up.
Izzie - Oh. And bring out this giant blow torch. My goodness!
Dave - So I’ll now heat this up to kind of orange hot.
Izzie - And I’m going to take a step back! Okay. So orange hot isn’t exactly a temperature we all understand. Dave heated this lump of neodymium iron boron to temperatures about 800 degrees celsius. And yeah, it started to glow…
Dave - I’ve now put that down next to a very strong magnet and let it cool down slowly.
Izzie - But how does cooling a lump of hot metal near a magnet then turn it into a magnet itself?
Dave - Because it’s really hot, these magnetic domains can twist round very easily. And then, as it cools down it becomes harder and harder for them to spin round so, hopefully, you’ll kind of ‘freeze in’ the magnetic field which it sees now.
I’ve now moved this piece of neodymium iron boron away from the magnet and I’ve put it next to a compass, and if I spin it round, the compass turns round.
Izzie - Oh wow! So we have just literally taken something and turned it into a permanent magnet?
Dave - Yeah. And because it’s neodymium iron boron this is now a very permanent magnet, and it will stay like this for years and years and years.
Izzie - So would this method work with say the Earth’s magnetic field?
Dave - You’d get a much much weaker magnetisation but you do get effect. So it’s really important in geology because if you get a molton piece of rock it’s basically like heating it up to red hot, and if you’ve got any little lumps of magnetic material in there, as it cools down they’ll freeze in the Earth’s magnetic field, which was there when it was created. And that can tell you stuff about the Earth’s magnetic field over time because if you know how old the rock is you know what the Earth’s magnetic field was like.
Izzie - So is the Earth a giant permanent magnet?
Dave - The short answer is no, and I can prove why it can’t be. What I’ve got here is an iron nut again next to a magnet - t sticks really quite effectively. Now what I’m going to do is heat this up.
Izzie - And it’s back to the blow torch. Our Earth’s core is full of iron. We took this tiny iron bolt and heated it to, again, 800 degrees celsius. So we can use heat to turn certain metals into magnets, but it can also demagnetise them.
Dave - And as I put it near the magnet it doesn’t stick at all. As it cools down eventually it does start to stick.
Izzie - Oh gosh, yes - it’s just stuck to it. So what's going on here?
Dave - If you heat up a ferromagnet like iron hot enough you give it enough energy that the individual atoms stop linking to each other and they just start to randomise. And that’s all pointing at completely random direction so it stops being even magnetic at all. This happens at a temperature called the Curie temperature. Then, as it cools down, they start lining up again and it starts becoming a magnetic material again.
And so, this can prove that the Earth can’t be a permanent magnet because Curie points may be a thousand, maybe slightly above that, degrees celsius and the center of the Earth is much much hotter than that so it can’t possibly be a permanent magnet.
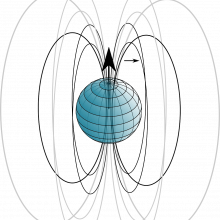
37:31 - Why is the earth's magnetic field important?
Why is the earth's magnetic field important?
with Professor Kathy Whaler - The University of Edinburgh
What has the earth's magnetic field ever done for us? Chris Smith put this to Kathy Whaler from the University of Edinburgh. First off, Chris asked Kathy what shape the field is...
Kathy - Well interestingly, the shape is very much like a bar magnet as we’ve just been hearing with two poles - a north pole and a south pole. And because the convection, the liquid is moving around so strongly influenced by the Earth’s rotation, that bar magnet field lines up with the north-south poles approximately. So it does look very much like a bar magnet but it’s caused by a very different mechanism.
Chris - What benefits does the field impart to us as dwellers on Earth?
Kathy - Well, we’ve already heard about it shielding us from the vagaries of space weather so it stops solar charged particles reaching the Earth’s surface for most of the part. It gives us a way to navigate as you were saying at the start of this programme. It imparts a signature to the geology which we’ve found helpful in exploration for resources. And it really is our protective shield and that’s perhaps the most important thing about it.
Chris - And in protecting us that also gives us that lovely light show that we call the Aurorae?
Kathy - That’s right.
Izzie - Why is it so important to study it and what can it tell us?
Kathy - Well, it does provide our window on the core, so a lot of the information that we have about the Earth’s core has come from knowing its magnetic field and knowing how that field changes over time. We can also use it investigate the geology; we can look for mineral resources; we can look for hydrocarbon resources - it’s used to help when we’re drilling for those resources. We can even see a magnetic field in the oceans when we’re looking at the data collected by low Earth orbiting satellites. So because the oceans are a salty water, as they move around they also generate a magnetic field in a rather similar way to the Earth’s core itself. And we can see the ocean tides even.
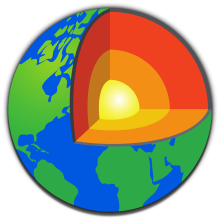
39:52 - Ancient magnetism and flipping the field
Ancient magnetism and flipping the field
with Professor Richard Harrison, Cambridge University
If you take a potentially-magnetic material at high temperature and cool it down in the presence of a magnetic field, it remains magnetised. And this also happens on Earth, when molten rocks from the planet’s interior solidify at the surface; as they do so they capture a snapshot of the magnetic field in which they formed, which is how we know the Earth’s magnetic field has flipped around in the past. Chris Smith spoke to Richard Harrison, who studies this at Cambridge University. First up, Chris asked Richard how he reads the signatures within the rocks he studies...
Richard - Well, first of all you have to collect some samples. A paleomagnetist who studies these ancient magnetic signals will go out into the field with something like a modified chainsaw, drill into the rock, extract an oriented core - we know exactly what orientation it was taken out of the Earth - take that back to a laboratory.
You then have to measure its magnetisation so we have extremely sensitive magnetometers, usually called the SQUID magnetometers - that Superconductive Quantum Interference Device. They’re able to detect the really weak magnetisation of these things. And we put that into what’s called a shielded room so we want to measure the magnetisation in the absence of the Earth’s field. So we build these shielded rooms; they’re big wooden structures lined with metal, a little bit like a Faraday Cage for magnetism. It shields out the Earth’s field so we can measure it in a zero field environment and see what the magnetic memory of that rock is.
Chris - Does that tell you though how strong the magnetic field is because that’s the other important component isn’t it? Not just what direction it’s pointing in but also how big it is?
Richard - Yes. So we get two fundamental pieces of information from these rocks. We can tell the direction of the field that was present when the rock erupted and cooled at the surface, but we can also tell how intense the magnetic field was. And we can trace both of those things back through time by looking at rocks with lots of different ages.
Chris - You gave me a very nice sample when we came in - this little crystal and a very strong magnet.
Richard - Oh, yes.
Chris - What was that to show?
Richard - Okay. This shows why rocks are magnetic in the first place. And they’re magnetic because the contain a small proportion of a mineral called Magnetite.
Chris - That’s this black crystal?
Richard - Yeah. So what you have in your hand there is a beautiful octahedron of magnetite, which is an iron oxide, and what you’ll find that if you place it close to that little bar magnet it should strongly attract it to it.
Chris - So this is basically a chunk of rock and I’m going to bring it close to the magnet and it just leapt off my hand and stuck to it. So basically, you’re recording tiny signatures written into minerals a bit like that one when you do your experiment?
Richard - That’s right. Magnetite makes a very nice magnet if the particles are small enough. There’s this sort of Goldilocks zone for particle size so that piece you have in your hand is millimetres in size, that would be too big, it doesn’t have a very good memory. But if we shrink those particles down to just a few hundred nanometers, they become excellent magnets with a really good memory of the Earth’s magnetic field.
Chris - And what have you learned about Earth’s geology, the evolution of our magnetic field, and the evolution of the surface of the Earth by actually studying these signatures?
Richard - Well, paleomagnetic measurements have been absolutely fundamental in our discovery of how the Earth works. The theory of plate tectonics really came about through studying the magnetic signals in the ocean floor. And through studying the magnetic memory that you see there it was able to prove that the ocean was spreading and that continents were drifting across the Earth.
Chris - Why the ocean floor? Why is that critical?
Richard - During the Second World War, people mapped out the ocean floor magnetically in detail. They wanted to detect submarines so they needed to map out the magnetism of the ocean floor. And what they found were these magnetic stripes that go parallel to a ridge of volcanoes all the way down the centre of the ocean. The magnetic stripes are symmetrical either side of the ridge and what they track over time is this random flipping of the Earth’s magnetic field where the north and the south poles switch around.
Chris - And they’re symmetrical either side of the ridge because what, the sea floors being born there and moving away?
Richard - Yeah.
Chris - If it’s parallel to the ridge that’s because the sea floor was born on each side of the ridge at the same time so it inherited the same field at that moment in time?
Richard - Exactly. So the magnetism is recorded as that new ocean crust in the centre of the ridge is cooled, and it would cause the present day field and then it’s pushed aside to both sides. And then later on the Earth field flipped and you get a different direction being recorded at the centre of the ridge. So that pattern of magnetisation was absolutely critical to determining that continental drift was real and that led eventually to the revolution of plate tectonics.
Chris - Can we use the same trick, Richard, with not just samples from Earth but other planets? We have meteorites from Mars, for example. We have samples of lunar rock. Can we look at magnetic fields in those?
Richard - Absolutely! A lot of the work we do in Cambridge is looking at extraterrestrial materials. So that could come from studying meteorites where we can look at the magnetic fields generated by asteroids early on in solar system. We looked at magnetism during the Apollo missions on the Moon and we were able to bring rocks back from the Moon.
Chris - The Moon does have a magnetic field doesn’t it?
Richard - It doesn’t have one now but it did have about four billion years ago. Early on its formation - yes.
Chris - So where’s it gone then? Why’s the Moon no longer got one?
Richard - Well, the Moon has a very small core. As in the case of Mars, the conditions have to be just right for that core to generate a magnetic field. When you have a smaller body that’s cooling quickly, the dynamo tends to switch off at some point.
Chris - You mentioned earlier about the field flipping and things. People are often quite curious about this. Has that happened lots of times in the past and when’s it due to happen next? Should we be worried?
Richard - We shouldn’t be worried. The Earth’s field has flipped many many times in the Earth’s past . It flips, on average, something like three to five times per million years. The last reversal was 780 thousand years ago. Life has persisted through all of those reversals so there doesn’t seem to be any majorly dangerous impacts of having the Earth’s field flip. The main thing you’ll notice is that mobile phone signals will get worse when there’s a flip.
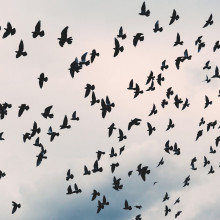
46:11 - Can birds see Earth's magnetic field?
Can birds see Earth's magnetic field?
with Professor Peter Hore, University of Oxford
Apart from being very useful for geologists who want to understand the planet’s past, as well as fending off the onslaught of the solar wind, and helping humans who want to navigate the old-fashioned way - with a compass - Earth’s magnetic field is essential to many migratory species: birds appear to be able to read the direction of the field and use it to guide them as they fly thousands of miles. Francesca Fazey spoke to Oxford scientist Peter Hore who suspects that proteins called cryptochromes in the birds’ eyes enable them to “see” magnetic fields...
Francesca - Close your eyes and turn around five times. Now, without opening your eyes head towards magnetic north.
Okay. So you don’t actually have to try this. And please don’t especially if you’re listening near a busy road.
But if you were able to do it, chances are you’d be a robin or any other kind of migratory bird. Scientists have worked out that birds that migrate can actually sense the direction of the magnetic field using some neat tests. Here’s Professor Peter Hore at the University of Oxford who specialises in this area…
Peter - The experiments involved testing the birds during the migratory season, so these are small migratory songbirds like robins. And during the migratory season in the spring and autumn, if you put them in a funnel shaped cage, the direction in which they hop to try and get out of the cages is the direction in which they would fly if they were released.
Francesca - Now, here comes the clever bit: using coils and currents you can change the magnetic fields that the birds are experiencing and then see if it changes the way they try and hop out of the cage and, incredibly, it did. So how do our feathered friends do this?
Peter - The leading hypothesis at the moment is that there are magnetically sensitive chemical reactions in the retinas of the bird’s eyes, and that these chemical reactions allow them to sense the direction of the field.
Francesca - A recent study has identified a specific protein with a great name - Cryptochrome 4 - that they think is the most likely candidate for a magneto receptor in these migratory species. This is because Cryptochrome 4, or Cry 4 as they like to call it, only seems to be expressed during the migratory season when they need it to guide their long journeys.
And get this: birds who don’t migrate, like chickens, don’t express Cry 4 at all. The protein is located in specific cells in the retina which are involved with vision. So does this mean the birds can actually ‘see’ the magnetic field? Peter has a classic scientists answer to this…
Peter - Maybe.
Francesca - So, no flight of fancy for us today then!
Peter - We don’t know nearly enough about how the signalling works. It is clear that the detection of the magnetic field involves the birds visual system, so the receptors are likely to be in the photoreceptor cells. We know that when the information from the retina reaches the brain it’s processed in a visual part of the brain. I think it’s too soon to say whether the birds literally see the magnetic field or whether it’s some visual impression which, of course, is a bit difficult for us to to imagine because humans don’t seem to have a magnetic sense.
Francesca - Although we have tried to find it. One study sent out blindfolded students into the woods and asked them to find their way out again. Amazingly they did without any serious injuries and the researchers suggested this was due to us having a secret sense of magnetic north much like the migratory birds. But, like many of these findings, it has never been replicated so we can’t genuinely claim to have this superpower.
Some other animals though might…
Peter - There was a high profile study a few years ago in which the scientists analysed Google Earth images of herds of wild deer and domestic cattle and found that the animal’s body axes tended to align with the Earth’s magnetic field.
Francesca - Gosh!
Peter - That proved difficult to replicate, but it’s a hint at least that maybe these land animals that do migrate large distances might have some use for the Earth’s magnetic field.
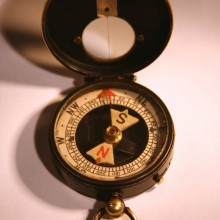
51:20 - Bacterial compasses - can creatures detect magnetic fields?
Bacterial compasses - can creatures detect magnetic fields?
with Professor Richard Harrison - Cambridge University
It’s not just birds that are sensitive to the Earth’s magnetic field. There are lots of animals and other organisms that are emerging now as being sensitive to magnetism. Chris Smith put this to Richard Harrison from Cambridge University...
Richard - Yes, that’s right. And one of the best known examples of an organism that uses the magnetic field to navigate is in a type of bacteria we call magnetotactic bacteria. And these are amazing little creatures that have learnt to build chains of magnetite nanoparticles inside their cell, which essentially turns that bacterium into a compass and they swim along the Earth’s magnetic field lines so that they can find, very efficiently, the correct level in the water column for them to live.
Chris - I was going to say, what would be the advantage to a bacterium to be able to sense the magnetic field?
Richard - Well, they live under very specific conditions. They need: too much oxygen would be poisonous to them, too little is again not ideal so they live at the redox boundary between the two. If you swim around randomly trying to find that level it can be a very inefficient way, but if you orient yourself in the field and swim in an exactly straight line then you get there very efficiently.
Chris - I read a story in the journal eLife. About three years ago researchers published a very interesting paper on tiny microscopic worms, and they found that these worms would swim in a certain direction through the jelly they grow them in when they’re in England. But if you send them to Australia, to Adelaide where they did these experiments, the worms will swim in the opposite direction.
And studying the worms, it turns out that there is a set of nerve cells at the back of the worm which are very long in one direction but very narrow in the other axis. And they think they behave a bit like an antennae, which means they might be sensitive to the inclination of the Earth’s magnetic field. Because, if you also do the experiment and grow these worms in an applied magnetic field, you can change this behaviour. And if you abolish those nerve cells that are in the worms they lose the ability to detect magnetic fields. So it looks like they have evolved a way independently to have their own magnetic antennae inside the worm, again, to find the right level for where they need to feed.
Richard - Yep, that’s very interesting. And the idea there is in the northern hemisphere the inclination of the Earth’s magnetic field is pointing down towards the ground, but if go into the southern hemisphere the Earth’s magnetic field is pointing up into the sky. So if they’re sensitive to that change in orientation then that might affect the way that they swim.
Chris - Be tricky for them if they went to Mars then. As we were hearing from Kathy earlier that Mars has lost its magnetic field, things like that just wouldn’t work would they?
Richard - Well, Mars did have a magnetic field early on in its history. And there are the infamous claims of evidence for these magnetotactic bacteria in the Allan Hills ALH84001 meteorite where people have found the magnetite nanocrystals which are remarkably similar to those that we find in Earth-based bacteria. But I should say, not everyone believes that theory.
Chris - And talking of people believing it or not, there are people also working on whether humans have these abilities, and we just ignore them because we have more dominant skills like our eyes and a GPS so we tend to suppress these other potentially latent magnetic-sensitive skills.
Richard - Yes. I know people who are working on that and they’re finding some interesting results. Unfortunately, I’m not allowed to talk about it because it’s top secret research, but we can say that there is some evidence pointing towards the fact that large primates, should we say, might be able to sense the magnetic field.
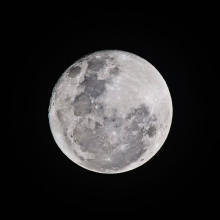
55:00 - Can we terraform the moon?
Can we terraform the moon?
Tamsin Bell asked planetary scientist David Rothery from the Open University to weigh in on this question from Chad.
Is it possible to terraform the Moon so humans could live there long term?
Tamsin - We asked our followers on the forum what they thought. User, diverjohn points out that the Moon is relatively close to Earth and suggest living in underground spaces would be possible. We could even use solar panels on the Moon’s surface to supply our electricity.
I spoke to Planetary Geoscientist David Rothery from the Open University…
David - Terraforming is usually understood to mean modifying the atmosphere, and hence also the temperature of a body to give it an environment where we could live. So in terms of us going to live in the open on the Moon, the answer is no. Because the Moon’s gravity is too weak to hang onto relatively light molecules such as water vapour, oxygen, and nitrogen in the long term.
Even supposing you found a way to liberate enough of those gases at the Moon’s surface to give it a breathable atmosphere, you’d have to continually replenish it as it leaked away to space.
Tamsin - So like all ‘bad first date’ restaurants on Earth, restaurants on the Moon would have no atmosphere…
David - It’s not just the Moon’s weak gravity that’s the problem, the Moon has no magnetic field to deflect the solar wind so this would always be eroding the top of the atmosphere.
The closest we might one day come to to a terraformed environment on the Moon might be inside a large transparent and leak proof dome. Fill this with the right mix of oxygen, carbon dioxide, water vapour, and nitrogen to more or less match the Earth’s atmosphere, and you could probably arrange for the average temperature inside the dome to be comfortable for humans, despite the nights lasting for two weeks. You could probably grow crops there too after a lot of work on the soil to get its structure and the microorganisms right.
Tamsin - If we did decide to move to the moon, we certainly would need to planet …
David - The biggest obstacle to humans living on the moon, or indeed anywhere in space, is the cost of transporting whatever resources you need from the Earth. Things would be so much easier and cheaper if we could get most of what we need at our destination rather than taking it with us.
We know there’s water in the form of ice inside the shadowed craters near the Moon’s poles. There’s plenty of oxygen that you could liberate at a large energy cost from lunar rocks. Carbon and nitrogen might not be so easy to come by though so we might need to depend on the Earth for quite a while.
Tamsin - Thanks for bringing us back down to Earth, David.
Next time we’ll be sticking to this one from Tom in Australia: Why is Blu Tac sticky?
Comments
Add a comment