It's big, it's blue, it's where life began and life certainly wouldn't be the same without it: yes, that's right, it's the sea. This week Helen Scales is taking the show underwater to explore her favourite realm. Among the marine menagerie she'll be revisiting the incredible story of squid that see with their entire body, once again be meeting the humming toadfish, which is teaching us a thing or two about making music, and we'll catch up with the colourful clownfish that, just like Nemo, might soon be needing some help finding their way home...
In this episode
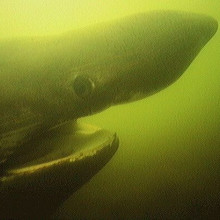
Giant shark mystery solved
Basking sharks, the second largest sharks in the world, have been tracked on epic, thousand-mile migrations into the deep waters of the West Atlantic, solving a long-standing mystery of where they spend the winter. Gregory Skomal from the Massachusetts Division of Marine Fisheries in the US led a team of scientists who tagged 25 basking sharks off the coast of New England.
As the satellites began beaming information back to the team they were amazed when the sharks kept on swimming south, into the Caribbean Sea and beyond. One shark even crossed the equator ending up at the mouth of the Amazon River on the Brazilian coast where it hung around for a month.
Their discovery has revolutionised our understanding of these mysterious basking sharks which until now were thought only to live in temperate waters. They have never been seen in this part of the world before, probably because they swim very deep down between 200 and 1000 metres.
The obvious explanation for their migrations is that these cold-blooded sharks need to find warmer water with lots of plankton, their favourite food. But what puzzles Skomal and his team is why the sharks bother going so far south. If food and warmer water were all they were after they could stay in northern Florida. Why bother going all the way to Brazil? One idea they came up with is that the sharks are moving to as yet undiscovered birthing and nursery grounds.
Amazingly, scientists have never seen a young or embryonic basking shark, so we know virtually nothing about how they breed. Previously it was thought that basking sharks form many separate sub-populations but now it seems they are well connected and could form a single ocean-wide population. That raises important issues of how we protect them, since any impacts on basking sharks in one area could have affects on the population as a whole. It could be nations around the world will need to get together and create a global basking shark conservation programme.
And this study just goes to show just how much more we still have to learn about some of the biggest creatures that roam the oceans today.
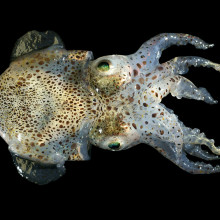
Squid don’t just see with their eyes
Squid, those slippery denizens of the deep, may not only see through their enormous round eyes but it seems they can also detect light all along their bodies as well.
Researchers from the University of Wisconsin-Madison have been studying the Hawaiian bobtail squid. These 3cm long squid have ink sacs on their bellies that don't just squirt out ink but also glow - a process known as counterillumination. When predators look up at the squid from below, the outline of the squid doesn't show up as a dark silhouette but instead blends into the bright background of the oceans' surface. Margaret McFall-Ngai and her team publishing in the journal PNAS have discovered that these ink sacs are capable of not just emitting but also detecting light.
A type of bioluminescent bacteria called Vibrio fischeri live inside the squid ink sacs in a two-way symbiotic relationship that benefits both squid and bacteria. The bacteria help the squid camouflage themselves against the bright ocean surface and in return, the bacteria get a safe place to live with all the nutrients that they need.
The bacteria emit a constant glow but the squid can control how much of that light escapes by changing the shape of the ink sac tissues, rather like the iris can let more or less light into an eye. The squid ink sac even has a transparent layer across the surface that acts like a rudimentary lens controlling the direction of the emitted light. By letting more or less light through from the bacteria, squid can match themselves to the brightness of the sea surface that varies depending on how deep down they are and what time of day it is.
The question is, how do the squid know how light the ocean is around them? It now seems that they might not only use their eyes, but the ink sacs also have a role to play in detecting ambient light.
McFall-Ngai have discovered that the ink sack tissues housing the luminous bacteria contain genes that produce proteins associated with light detection, including some similar to ones found in the retina - the light detecting layer of cells in the eye. They also hooked up an ink sac to an electroretinogram - electrodes that are usually used to measure the electrical responses of a retina when light is shone on it. They found similar electric signals were generated by the squid ink sac, indicating that it is sensitive to light.
The researchers don't yet know exactly how these second light-receptors evolved. It could be a form of genetic or evolutionary "tinkering", a technical term in which existing components of a living system are reassembled and tinkered and put to use in new combinations or locations. More studies are needed to delve deeper into exactly what is going on.
This study sheds light into a remarkable symbiotic relationship between bacteria and large animals, something that is particularly important for us humans to understand since we rely on trillions of bacteria living inside us to keep us healthy. We may not glow brightly at night, but eight out of ten of our major organs have some sort of bacteria living in them.
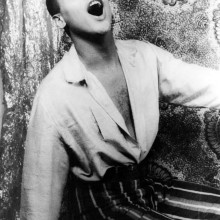
From a fish that hums to Pavarotti
If you like to warble in the shower or in the bath then you probably don't realise that you are in fact taking a step closer to the origins of your singing talent, namely fish.
That's according to a team of neuroscientists from Cornell University in the States led by Andrew Bass, who have discovered that vertebrate brains may have been wired up for making sounds for a very long time: hundreds of millions of years ago, in fact, since a time before vertebrates hauled themselves out onto dry land.
The team studied the brain development of a type of fish that lives on the seabed along the Pacific Coast of North America called the midshipman or - I think rather wonderfully - the humming toadfish. Male humming toadfish spend long hours humming happily away, serenading females and trying to tempt them to lay their eggs.
They injected fluorescent dyes into the growing brain cells of toadfish larvae and watched under microscopes as clusters of cells formed connections and eventually grew into networks that control the fish's vocalisation.
By looking at the equivalent brain the team discovered remarkable similarity with the neural circuits controlling sound-making in amphibians, birds and mammals. This bolsters the idea- first put forward by Charles Darwin - that the ability to make and control sounds first evolved a very long time ago in the ancient ancestors of all modern day vertebrates when they were still swimming around the sea.
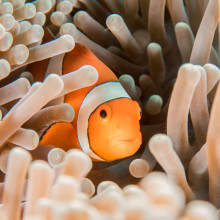
Nemo could get lost in acidic ocean
If you've seen the movie Finding Nemo, then you'll know that Nemo the clown fish got lost and had to try and find his way back to his home reef.
Now it seems that the Disney animators may have been onto something, because a study published in the journal PNAS led by Philip Munday from James Cook University in Queensland, Australia, found that clown fish may indeed get lost if the oceans become more acidic. And that is likely to happen as more carbon dioxide enters the atmosphere and dissolves in the seas forming carbonic acid.
Many coral reef fish spend the first few weeks of life as tiny larvae drifting through the open ocean. And previous studies have shown that they follow their noses and their ears, sniffing out and listening to sounds that lead them back to the reefs they were born on.
But, it seems that as the acidity of seawater increases, fish may loose their sense of smell and have trouble finding their way home.
Munday and his team took newly-hatched clown fish larvae and put them in choice chambers in the laboratory filled with water containing different chemicals. In seawater of normal acidity, the clownfish preferred to swim in the plume of water that smelt of rainforest trees, because in the wild they live on reefs that surround vegetated islands.
When the acidity was increased, the clown fish instead choose to swim in the plume of water that smelled of swamps, a smell they hate and usually avoid.
It may not sound like much of a difference - swamps or rainforest trees - but if wild fish start to loose their ability to find the right sort of habitat, it could spell disaster for entire populations and ecosystems.
So this study spells out yet more gloomy forecasts for the changes that might take place as carbon dioxide continues to build up in the atmosphere and even more reason to try and find ways of curbing our emissions of the greenhouse gas.

Fizzy Acids - what happens when you carbonate water
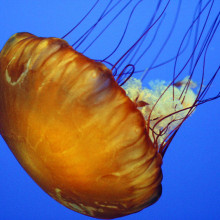
19:38 - Keeping Tabs on Jellyfish
Keeping Tabs on Jellyfish
with Jon Houghton, Queen's University Belfast
Ben - We now have John Houghton on the line. Thanks very much for joining us. You are studying turtles so why is it that you're tagging jellyfish?
Jon - Leatherback turtles are the ones that eat jellyfish and they cause a bit of a problem for us because they're not like a typical migratory species that moves from one spot to another. They just fan out through the entire ocean. We, for a long time, haven't actually known where they were feeding or what they were feeding on. [For] a couple of years we did big surveys of the whole Irish Sea. What we found was not what we thought we were going to find. We thought the jellyfish were just going to be randomly everywhere. What we found was in four or five main bays. You get these hundreds of thousands of giant jellyfish that are there year after year after year. When we modelled the distribution of leatherback turtles we actually find they're tied up in the same place. That wouldn't be very exciting if you work on land but when you work on an animal that lives beneath the sea and you can never blimmin' find it actually just a simple thing of tying predator and prey is very good.
Ben - How do these electronic tags work? I'm guessing these are not the things that report you're not in your home when you should be?
Jon - No but they're not a million miles away from it. They're data storage tags and they're tiny - they're about the size of your little finger. The ones we're gonna do this year are quite simple. They're just going to record depth and temperature and light levels. We just put it on to a jellyfish. It records all the information and then eventually we retrieve the tag.
Ben - I've seen plenty of jellyfish washed up on the beach. They're very squidgy sort of fluidy things. How on earth do you attach an electronic tag onto something that's so amorphous and blobby?
Jon - That's true but there's jellyfish and there's jellyfish. I think the ones you're describing would be called aurelias. They're common jellyfish. They're tiny and floppy and wobbly and they would be almost impossible to tag. The ones we're going after are called barrel jellyfish and they're massive. They're nearly a metre across and weigh 27-28kg. They are actually quite big, tough animals. They're very strong swimmers, they can swim against a current. Actually if you think your jellyfish are looking like a mushroom. You've got the stalk part coming out underneath what we call the bell. Quite simply all you do is you just tie a time and depth recorder to a plastic cable tie, swim up to the jellyfish: tie it around. It takes about ten seconds.
Ben - Does this affect their behaviour? They must not like having something stuck round their body.
Jon - They're very simple animals and they do react. That's true. We did trials off the West of Ireland last year. Not surprisingly, when you attach a tape to a jellyfish it just swims to the seabed and tries to get away from you. What we found, after an hour or so, they'll just move back up in the water and get on with jellyfish business. As long as you ignore those first few hours then it's fine. You're talking about a device that is 0.1% of the whole animal's body weight. It doesn't really affect it that much.
Ben - How long are they going to keep these tags on? When are you expecting to get this data back?
Jon - That we don't know the answer to. The particular jellyfish we're going after - they're unusual. Most jellyfish boom and bust for a couple of months in the summer. These guys seem to be around all year. We're going to put the tags on probably in August and I'm sure they could be turning up anytime between say two months to maybe even a year down the line. So yeah, any time over the next year.
Ben - How do you actually collect this data? Does it just float up from where the jellyfish were?
Jon - What we've got attached to the time-depth recorder, a little dive computer, is just a tiny fishing float. On the fishing float is just a little label with a reward on it. Once the jellyfish dies the whole device just detaches itself from the jelly and floats to the surface. We're putting them on in big bays where we know they will wash ashore. If you find one on the beach just pick up the reward label and give us a call.
24:36 - Robots of the Ocean
Robots of the Ocean
with Jules Jaffe, Scripps Institution of Oceanography University of California, San Diego
Jules - Over the past decade we've developed a rather large vehicle, something on the order of a Volkswagen Beetle-sized vehicle which has the capability of adjusting its buoyancy. On it we put laser and we put some very sensitive cameras. We put that off the ship. It weighs almost a tonne so it's hardly something you could go out on your row boat to put in the water! You need a fairly large ship. We've learned a lot of interesting things. The problem we kept coming up against (and it becomes more obvious) the more we process out data and the more we think about it is that we're just sampling one place at one time. Even though we get a nice picture from that place and there are inferences we can make; we're starting to work on distributed networks. Instead of having just one sampler in one place at one time we've got a small army of these things and they're all going up and down in the water. They're all sampling and they're all sampling different places at different times. If we know where they are and we know when they were there and we have the appropriate sensors on them we can start to reconstruct this three-dimensional distribution of these organisms and actually look at mechanism as opposed to just looking at, more or less, existence.
Chris - How do you get them to talk to each other? A major problem with underwater communication: as submariners know very well you can't get radio signals through water very easily. How do you keep them in touch with each other?
Jules - The ocean, as you've already highlighted, is particularly opaque to electromagnetic radiation. Acoustics, on the other hand, as has been known by animals that have evolved over 100s of millions of years, can go very far. Our plan is to have these vehicles localising each other acoustically and we can communicate with one of them that is fairly close to us. They can distribute that message among the entire group by relaying that information. We think we can evolve sensor systems which can sense tens of kilometres instead tens of metres.
Chris - Would they be carried by ocean currents? You'd deploy a range of these things over a certain distance and the natural current would move them along?
Jules - Absolutely, we've actually had a wonderful insight into one of the advantages of our technology. We would like to be in the frame of reference of the organism itself. When you're sitting on a ship and you're bobbing up and down and you stick something in the water, that thing's going to be moving around. We found out a long time ago that it makes more sense to put the vehicle in the water and let it go with the flow, as we say, and to have maybe tens or even a hundred of these things. It allows us not only to take a snapshot of their evolving environment but also to track them. There are many enigmas in oceanography that we don't understand having to do with small animals that, say, are born onshore and have a pelagic part of their live where they go out to sea maybe for three months: a baby barnacle or something like that. Somehow they get back. To be honest, I don't have an idea how they do that. Most of the people I know who study these things don't have an idea how they do that! We can not only study this ten kilometre area but we can watch it evolve and be transported in time. Maybe start to unravel some of these riddles of how organisms survive in the ocean.
Chris - What you're building at the moment - is that going to be one of these vehicles?
Jules - That's correct. What we're looking at here, Chris, is something about the size of a soccer ball which has been cut in half. We're looking inside at the electronics of this device.
Chris - It looks like something that should be in Star Wars, actually...
Jules - There's a good story about that actually. In our last grant proposal to the national science foundation we wanted to put in a colour graphic of these devices. My colleague actually put the Death Star and shrunk it down. He created a picture where you have about 20 of these Death Stars communicating with each other. In fact we're not that far away.
Chris - Is that the right message to be sending out because you're actually trying to save the Earth rather than destroy it!
Jules - Yeah! Basically, we have this pretty simple thing. It's a soccer ball with a bunch of electronics inside it. The soccer ball's thick enough that we can send it down to about 80m of depth without imploding. The computer has sensors that tell it what the depth of the vehicle is. By adjusting its buoyancy (its volume in the ocean) we can actually send it down and keep it at a certain depth in the sea. What we also have on this vehicle: on the bottom here is an acoustic transmitter and receiver. We call that a transducer. It can send sound and receive sound. These are actually modems. They're actually communicating devices which allows these vehicles to talk to each other. They can actually range off each other. That allows us, knowing their depth and knowing how far they are from each other to estimate their 3D positions. Now imagine a fleet of maybe 100 of these things slowly descending in the sea, localising off each other; each equipped with some method of sensing plankton density or perhaps other things like zooplankton using acoustics. Using these hundred or so samples we can now create a 3D time-varying record of the evolution of a volume that might be 5km X 5km X 80m deep.
Chris - How long does it take you to develop something like this from concept to getting something that you can put into the pacific?
Jules - You've touched a very tender subject with me. You might have noticed I have a bit of comedy associated with my personality so what I tell my students is that if you're pregnant you're guaranteed within 9 months but if you're an engineer and you're building a vehicle it could be ten years. We're sort of giving birth. This has been an exciting week for us because ten years from the inception of this we've now started to test these things in the ocean on modem communication. It's really exciting but by the time - I tell our students that science is not something if you want immediate gratification - the time you think of the thing, write the proposal, perhaps get it rejected a few times before it's funded, do the research, get the results, process the data, publish it and go to colleagues and tell them what you've done it can often be a 7-10 year period.

32:55 - Trawling and the Damage Done
Trawling and the Damage Done
with Les Watling, University of Hawaii
Les - Trawling is a method of fishing that started in Britain in the 14th century where someone found out that if you took a net and held it open in some way and hauled it behind a boat you could get a lot of fish. You also get a lot of stuff. I've actually been looking at effects of fishing for about fifteen years and I've been in submarines and I've had cameras on remote vehicles. What I generally see is that where an area's been trawled there's not much living on the surface of the ocean floor. We know that trawlers also dig in to the sediment. They disrupt all kinds of things. Generally if you go to an area that's trawled it's really noticeable.
Meera - When the boats are going by what effects is the trawling having on the sea bed?
Les - Usually what happens is anything that's standing up from the bottom - anything like a sponge or coral that's growing up from the bottom - that's usually bent over, broken or removed. If it's a muddy bottom then the gear digs into the bottom. The important thing to realise is that most of the animals that live in the muddy bottom live in the upper 3 or 4 cm. You don't have to dig in very far before you've disrupted the burrows and tubes of all these small things.
Meera - They've been disrupted but what impact does that have?
Les - It depends. Some animals recover from this disruption okay, which means they can make a new burrow or two, but a lot of animals invest a huge amount of energy into making the burrow. In fact in some cases some of the marine worms, for example, they've lost the ability to remake a tube. Then that's it. They're laying on the surface and they could be eaten by a fish or any other thing that comes along. They've lost their protection, as it were. Other animals raise their young in their burrows and tubes. If that's destroyed then the babies may not be able to burrow their way out of this mud that's been disturbed. We tend to see species in these trawled areas that have really high reproduction. They're weeds in the best sense of the word. They have high reproduction, they can re-colonise. They're capable of getting their house blown down, if you want to think of it that way. They'll rebuild it real fast: all that sort of stuff. You tend to lose the things that have a longer, more stable lifestyle. Especially if it's an area that's trawled repeatedly. If a person drags a trawler over an area once then a lot of things will survive that. Maybe half of the things that live there will survive that. There will be a certain amount of re-colonisation that can occur in two years. A lot of times trawling occurs over and over again. Fishermen have their particular favourite spots. When you go to those areas you find that the whole bottom community has really changed to these weedy type species. This from a fish perspective might not be so bad. Fish can eat those weedy species to so you could get flat fishes, for example. It's been shown in the North Sea that if you re-trawl areas a lot you can get flat fishes but you might not get other kinds of fish because their food is missing. You create a completely different bottom community and a new ecosystem, as it were. One parallel that I like to use is what happens when you go in and, as happened in North America for example, colonists came. The cut down all the forest and they turned it into pasture land. So we lost all the birds that nested in the trees. It's a very similar kind of thing. The community's still productive for growing sheep or cattle or whatever but you have lost the outliers there.
Meera - How much of the world's marine ecosystem is being affected by trawling?
Les - That's a hard estimate to make. We looked at the number of fishing vessels and where they were around the world about ten years ago. We figured that about half of the continental shelves of the world get trawled each year. That number is obviously fluctuating because fisheries have collapsed in a lot of these areas. It may be the collapse of some of these continental shelf fisheries that will allow some of this biodiversity to recover.
Meera - What do you think the solution is? What can we now aim to do knowing this?
Les - This is going to be a little controversial but you know people have been proposing for a number of years to set aside and really protect the areas that you don't go into with any kind of gear. These areas have generally been proposed to be about 20-30% of the continental shelf area. We should be looking at it the other way around. We should protect most of the sea bottom and only allow trawls into a very small percentage.
Meera - I guess if that does happen do you think that the effects that trawling has had so far is reversible?
Les - It's reversible. The time scales are going to be long the deeper you go in the sea. I have a project that has just finished looking at an area that had been closed to trawling for six years. It doesn't look at all anything like the areas that have never been trawled. That's six years on. If you go into really deep water we know on the sea mounts, for example, that corals recruit at extremely low rates. We had a study where we were looking for coral recruits on the sea mounts of the North Atlantic and we found one on a block that had been put out for a year and a half.
Meera - So I guess fishing can be reduced but the outlook is quite promising. It's not something that's just going to be left disturbed.
Les - Promising in the long run. Yeah. For the continental shelves, for areas that have rocky-stony-marl bottoms those we're looking at 25-50 years probably for recovery time: before the big things like sponges start to re-grow. The deeper you go though the longer I'm certain that sea mounts that have had all the corals removed from the top will not have corals again for a century or two centuries. This is a really long time.
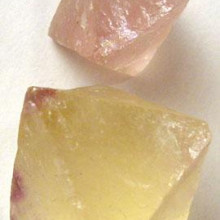
40:14 - Chemistry in its Element - Fluorine
Chemistry in its Element - Fluorine
with Kira Weismann, Zaarland University
The 37-year old technician spilled only a few hundred milliliters or so in his lap during a routine palaeontology experiment. He took the normal precaution in such situations, quickly dowsing himself with water from a laboratory hose, and even plunged into a nearby swimming pool while the paramedics were en route. But a week later, doctors removed a leg, and a week after that, he was dead. The culprit: hydrofluoric acid (colloquially known as HF), and the unfortunate man was not its first victim.
Unlike its close relatives, hydrochloric and hydrobromic acid, HF is a weak acid. This, coupled with its small molecular size, allows it to penetrate the skin and migrate rapidly towards the deeper tissue layers. Once past the epidermis, HF starts to dissociate, unleashing the highly-reactive fluoride ion. Free fluoride binds tightly to both calcium and magnesium, forming insoluble salts which precipitate into the surrounding tissues. Robbed of their co-factors, critical metabolic enzymes can no longer function, cells begin to die, tissues to liquefy and bone to corrode away. And if calcium loss is rapid enough, muscles such as the heart stop working. Burns with concentrated HF involving as little as 2.5% of the body surface area - the size of the sole of the foot, for example - have been fatal.
HF has a long history of destructive behaviour, claiming the lives of several chemists in the 1800s, including the Belgian Paulin Louyet, and the Frenchman Jérôme Nicklès. These brave scientists were battling to be the first to isolate elemental fluorine (F2) from its various compounds, using electrolysis. However, it was Nicklès' countrymen, Henri Moissan, who succeeded in 1886. To achieve this feat, Moissan not only had to contend with HF - the preferred electrolyte in such experiments - but fluorine itself, a violently reactive gas. His key innovation was to construct an apparatus out of platinum, one of the few metals capable of resisting attack, while cooling the electrolytic solution down to -50 °C to limit corrosion. Moissan's feat earned him the 1906 Nobel Prize in chemistry, but the celebration was short-lived. Another victim of fluorine's toxic effects, he died only two months later. Yet Moissan's method lived on, and is used today to produce multi-ton quantities of fluorine from its ore fluorspar.
Ironically, while elemental fluorine is decidedly bad for your health, fluorine atoms turns up in some 20% of all pharmaceuticals. The top-selling anti-depressant Prozac, the cholesterol-lowering drug Lipitor, and the antibacterial Cipro, all have fluorine to thank for their success. How is this possible? Because the flip side of fluorine's extreme reactivity is the strength of the bonds it forms with other atoms, notably including carbon. This property makes organofluorine compounds some of the most stable and inert substances known to man. Fluorine's special status also stems from the 'fluorine factor', the ability of this little atom to fine-tune the chemical properties of an entire molecule. For example, replacing hydrogen with fluorine can protect drugs from degradation by metabolic enzymes, extending their active lifetimes inside the body. Or the introduced fluorine can alter a molecule's shape so that it binds better to its target protein. Such precise chemical tinkering can now be carried out in pharmaceutical labs using an array of safe, commercially-available fluorinating agents, or the tricky transformations can simply be out-sourced to someone else.
Most of us also have fluorine to thank for our beaming smiles. The cavity-fighting agents in toothpaste are inorganic fluorides such as sodium fluoride and sodium monofluorophosphate. Fluoride not only decreases the amount of enamel-dissolving acid produced by plaque bacteria, but aids in the tooth rebuilding process, insinuating itself into the enamel to form an even harder surface which resists future attack. And the list of medical applications doesn't stop there. Being put to sleep is a little bit less worrisome thanks to fluorinated anaesthetics such as isoflurane and desflurane, which replaced flammable and explosive alternatives such as diethyl ether and chloroform. Fluorocarbons are also one of the leading candidates in development as artificial blood, as oxygen is more soluble in these materials than most other solvents. And radioactive fluorine (18F rather than the naturally-occurring 19F) is a key ingredient in positron emission tomography (or PET), a whole-body imaging technique that allows cancerous tumours to be discovered before they spread.
Fluorochemicals are also a mainstay of industry. One of the most famous is the polymer polytetrafluoroethylene, better known as Teflon, which holds the title of world's most slippery solid. Highly thermostable and water proof, it's used as a coating for pots and pans, in baking sprays, and to repel stains on furniture and carpets. Heating and stretching transforms Teflon into Gore-tex, the porous membrane of sportswear fame. Gore-tex's pores are small enough to keep water droplets out, while allowing water vapour (that is, sweat) to escape. So you can run on a rainy day, and still stay dry. Fluorine plays another important role in keeping you cool, as air-conditioning and household refrigeration units run on energy-efficient fluorocarbon fluids. And fluorine's uses are not limited to earth. When astronauts jet off into space they put their trust in fluoroelastomers, a type of fluorinated rubber. Fashioned into O-rings and other sealing devices, these materials ensure that aircraft remain leak-free even under extreme conditions of heat and cold. And when accidents do happen, space travellers can rely on fluorocarbon-based fire extinguishers to put the flames out.
Fluorine has long been known as the 'tiger of chemistry'. And while the element certainly retains its wild side, we can reasonably claim to have tamed it. As only a handful of naturally-occurring organofluorine compounds have ever been discovered, some might argue that we now make better use of fluorine than even Nature herself.
For more Chemistry in its Element, or the latest in Chemistry news from Chemistry World - visit the
Royal Society of Chemistry's Website.

53:38 - How do sharks smell blood underwater?
How do sharks smell blood underwater?
Thomas Breithaupt, University of Hull
Vince is absolutely right in questioning the scenario in wildlife programmes where sharks apparently are attracted from a distance within a very short time after some smelly substance has been dumped in the ocean. Water molecules in general are carried to the shark by water currents. If there are no water currents then it is molecular diffusion, the random movement of molecules that disperses the odour away from the source. Diffusion is an extremely slow process as Vince experienced in his ink experiment. In general the travel time of odour depends entirely on the local water velocity. Near the water surface water velocities in the ocean can range between a few centimetres per second on a very calm day and several metres per second in a strong current. In summary, odour can theoretically be detected by a shark in several miles from the source and I would estimate that in the ocean this may take at least one minute to reach the shark at a distance of 100m. More likely it will need between ten and twenty minutes. Finally the shark still needs to get to the source and that would take another 10-100 seconds depending on the swim speed of the shark. If smelly things are dumped into the ocean don't expect a shark to be attracted from a distance in less than a few minutes.
How do barnacles mate?
Helen Scales - You're talking about sperm and eggs, and barnacles if you've ever been down to this shoreline are rooted very solidly to the spot, so how do they move around and find a mate?
Well lots of other marine creatures have a similar problem. Things like corals which are animals, they don't move either but they solve that by sending their sperm and eggs up into the water and hopefully they'll fertilize and they'll meet each other and fertilization will take place and larvae will be created, but that's not what barnacles do. Barnacles look a bit like other types of mollusc on the seashore but they are in fact a type of crustacean, like crabs, and lobsters, and things like that, but very much smaller, but they do actually have sex directly and the only way they can do that is by having a very long appendage.
The male barnacles have very long penises, one of the longest in comparison to the size of the body, that there is in the animal world - animal kingdom, and - Chris Smith - They didn't include me in the analysis - I'd just like to say that.
Helen Scales - No comment - and the barnacle males, will literally poke around next to them and see what they can find, so they don't have much reach really in actual terms but they can reach out and fertilize female barnacles.
Chris Smith - That's amazing! So if you look at the barnacle crop that you see on rocks, do you see sort of concentric rings of males, females -?
Helen Scales - You must see some sort of patterning like that indeed, because you would have to be arranged in space, around on that rock to be able to reach other members of the opposite sex, and they obviously mate with lots of other different barnacles to produce lots of baby barnacles, and but that's how they do it - and that seems rather wonderful. So take a closer look next time you're down on the shore.
Chris Smith - Thank you very much, Helen!
Comments
Add a comment